Abstract
It is widely acknowledged that maternal nutrition impacts the health of offspring at birth. Less obvious is that maternal nutrition affects the fetal genome and shapes the health of the offspring well into adulthood. Epigenetics consists of changes taking place in a genotype due to external conditions, such as nutrition, that do not involve changes to the underlying DNA. The changes are due to the external conditions leading to the expression or silencing of genes in the genotype, and may have lasting effects during the life of offspring and into subsequent generations. Epigenetic effects occurring during gestation can lead to obesity in the progeny as well as reducing the resistance to diseases such as diabetes, cardiovascular dysfunction, and cancer. The tailoring of maternal nutrition is warranted to minimize the deleterious effects of overnutrition, undernutrition, or poor-quality nutrition. All three maternal nutritional models can lead to obesity in the adult offspring. While maternal obesity prior to conception contributes to obesity in the offspring, an adverse maternal diet will contribute to obesity without the mother’s obesity being a factor. Both a protein-restricted maternal diet, as well as a maternal high-fat diet (MHFD), can lead to obesity and cardiovascular disease in the offspring. Developmental plasticity also plays a role as the epigenetic effects of the maternal diet create the “expectation” for a matching postnatal nutritional environment. In addition, maternal diets, when including key nutrients, present beneficial epigenetic effects in cancer prevention. Tailoring a maternal diet to avoid epigenetic pitfalls, excess weight gain, the stereotypical Western diet, and including key nutrients will lead to healthier fetal development that continues into adulthood and future generations.
Where did epigenetic studies originate?
The early beliefs about human traits were that they were fixed from conception. This belief yielded to Charles Darwin’s theories that proposed that changes are possible through the influence of environmental factors, thus introducing the concept of evolution. Gregor Mendel’s studies of pea plants circa 1865 provided the systematic framework to understand how traits transfer from one generation to the next through inheritance. Subsequently, the DNA molecule was discovered in 1869, but its double-helix structure was not revealed until the next century. With a greater understanding of DNA, the principles of genetics and the role of DNA in heredity were uncovered (Peixoto et al., 2020).
Developmental biologist, Conrad Waddington, used the term “epigenetics” to describe the environmental links to gene and protein expression and to capture the concept that a single genotype could produce multiple phenotypes (Waddington, 1942). The rapid expansion of epigenetic knowledge starting in the 1980s led to the understanding that gene expression or silencing can result in either beneficial or harmful conditions. The importance of environmental factors in gene expression is still incompletely understood, and these factors are the subject of extensive contemporary research. A significant portion of this research investigates how nutrition impacts gene expression during pregnancy and the epigenetic results in the offspring and the ensuing generations (Peixoto et al., 2020).
Why are epigenetic effects an issue?
Epigenetic research studies how environmental and lifestyle factors, such as climate, diet, and water intake, affect the expression of a person’s genes and production of their structural and functional proteins. An important difference to note between epigenetic changes to DNA and genetic mutations is that epigenetic changes are reversible and do not affect the base genetic sequence. As epigenetic effects possess the potential to influence gene expression and subsequent health outcomes across generations, an understanding of their mechanisms and implications is crucial for effectively addressing health challenges and informing preventive strategies for the future.
While research is pursuing a greater grasp of the broad field of epigenetics and its ever-expanding list of interrelated gene-influencing mechanisms, the societal view of the maternal diet has failed to focus on its longer-term epigenetic consequences. The most well-known consequence is the globally-acknowledged problem of obesity, but other significant consequences include diabetes, cardiovascular disease, and some cancers. For many years, it has been known that environmental factors throughout gestation (e.g., maternal nutrition, maternal lifestyle, metabolic diseases) may cause changes in gene expression and permanently damage the structure and function of some specific organs of the fetus, most notably the liver and kidneys (Harmancıoğlu and Kabaran, 2023). While continued research is required to strengthen direct cause-effect relationships, substantial evidence links post-translational modifications such as DNA methylation and histone modifications of several candidate “obesity” genes to the predilection for obesity (Smith et al., 2023). Furthermore, modern biology uses epigenetic changes as molecular tools for finding and treating various diseases including cancer. Cancer is a multi-step process derived from combinational crosstalk between genetic alterations and epigenetic influences through various environmental factors (Meeran et al., 2010). Subjugation to maternal obesity, famine during gestation, nutritional supplements, drugs, alcohol, or other chemical agents can trigger epigenetic alterations that impact the development of the embryo and placenta (Smith et al., 2023).
The purpose of this study is to provide a scientific foundation that highlights the need for attention to maternal dietary practices that minimize negative epigenetic factors while including epigenetically favorable, even curative, dietary components. Following a healthy diet during pregnancy is a well-known practice. In much of society, however, this has taken on the status of an “old wives’ tale” and should be reconnected with old and new scientific research to expound on the true impact of unhealthy maternal nutrition. The interplay between nutrition, epigenetics and lifestyle in early life requires further research and self-advocacy to understand risks and control consumption and health impacts. Public health issues, by some thought to be due to individual lifestyle decisions, can be improved, treated, and/or prevented by addressing their epigenetic origins.
Literature Review
Overnutrition, undernutrition, and poor-quality nutrition (chemicals, preservatives, contaminants, Western diet, inadequate nutrients) are the three most common adverse nutritional pathways followed during pregnancy. There is growing evidence that maternal nutritional status can alter the epigenetic state of the fetal genome, which implies that there are stable alterations of gene expression through DNA methylation and histone modifications. For example, individuals exposed to the Dutch winter famine of 1944–1945 in utero had higher rates of insulin resistance, vascular disease, morbidity, and mortality in adulthood (Bazer et al., 2004; Lumey, 1998).
Epigenetic alterations in early embryos may be carried forward to subsequent developmental stages (Waterland and Jirtle, 2004). Two of the mechanisms mediating epigenetic effects are DNA methylation, occurring in multiple positions of cytosine residues throughout the mammalian genome, and histone modification (Bazer et al., 2004; Jaenisch and Bird, 2003). Histone acetylation or methylation can alter the positioning of histone-DNA interactions and the affinity of histone binding to DNA, thereby affecting gene expression (Bazer et al., 2004; Jaenisch and Bird, 2003).
Obesity
Worldwide obesity has nearly tripled since 1975 with currently over 1.9 billion adults overweight or obese (WHO, 2018). Obesity is a recognised global problem and was declared an epidemic by the WHO in 1997. It, therefore, represents a significant health and economic burden to societies, with 5% of deaths worldwide in 2013 being attributed to obesity (Dobbs et al., 2014; Inzani and Ozanne, 2022).
Strong associations between aberrant DNA methylation and various disorders such as neuropathies, malignancies, and metabolic dysfunctions, such as obesity, are reported (Smith et al., 2023; Al-hussaniy et al., 2022). These findings further support the existence of a relationship between the obesity phenotype and the methylation status of genes that control the body’s desire for food and, therefore, food intake (Smith et al., 2023).
Within the context of epigenetic influences on obesity, long non-coding RNAs (lncRNAs) and microRNAs (miRNAs) are considered among the most relevant non-coding RNAs studied (Mahmoud, 2022; Smith et al., 2023). LncRNA molecules have been implicated in obesity to the degree of their regulatory function in the metabolism of lipids, glucose, and cholesterol. Epigenetic factors affecting the function or bioavailability of lncRNAs and miRNAs can therefore have an impact on metabolism and either contribute to the development of obesity or act as biomarkers or therapeutic targets for obesity. Epigenetic modification of lncRNA betalinc1 may make a person susceptible to either hypo- or hyper-insulinemia, hyperglycemia, and insulin resistance, which can lead to obesity (Smith et al., 2023). Hypoinsulinemia is a low amount of insulin in the bloodstream and is often associated with type 1 diabetes. Hyperinsulinemia is a higher than healthy amount of insulin in the bloodstream and is often associated with type 2 diabetes. Hypoglycemia is low blood sugar (glucose) and is often associated with type 1 diabetes (NIH.gov, 2024).
Catherine Phillips, the principal investigator and coordinator of the ALPHABET project said: “Previous research has suggested that lower maternal carbohydrate intake in early pregnancy can induce epigenetic changes – that is, changes which alter gene expression – in children that may be associated with an increased risk of obesity”. They propose that a lower quality maternal diet, high in inflammation-associated foods, may similarly induce epigenetic changes and that this may increase the risk of children having obesity or excess body fat in later childhood (News-medical.net, 2021). Also noted is that human growth restriction early in gestation increases the probability of developing obesity in later life, particularly if combined with rapid compensatory growth after birth (Ando et al., 2023).
There is evidence of altered adipocyte metabolism and increased fatty acid accumulation in fetuses of obese pregnancies, with increased expression of fatty acid, glucose transporters and of fatty acid biosynthesis enzymes in fetal sheep adipose tissue in response to maternal obesity. This is accompanied by altered expression of genes which regulate adipogenesis, lipogenesis and the synthesis of adipokines, leading to increased lamb fat mass observed in early postnatal life (Inzani and Ozanne, 2022).
Over the past 30 years, there has been an increased omega-6/omega-3 polyunsaturated fatty acid (PUFA) ratio in human breast milk, correlating with heightened maternal consumption of refined vegetable oils rich in omega-6 PUFAs. Indeed, changes in breast milk fatty acid composition associated with maternal high-fat feeding have been linked to DNA demethylation in genes expressed specifically in white adipose tissue. Recent findings highlight the role of human milk oligosaccharides (HMOs) in reducing infant adiposity, suggesting their potential in protecting against obesity when breastfeeding. Unlike infant formulas, HMOs are abundant in human milk and may function as prebiotics, potentially aiding in reducing fat build-up by fostering healthy gut microbiota and their resultant metabolites like short-chain fatty acids (SCFAs) (Smith et al., 2023).
In addition, histone acetylation with respect to tumor necrosis factor (TNF) and the resultant insulin resistance may encourage the emergence of the obesity phenotype. Interestingly, caloric restriction is reported to revert the increase in acetylation to the normal type. This finding lends further support to the notion that the modification of TNF is epigenetically regulated (Mahmoud, 2022; Smith et al., 2023).
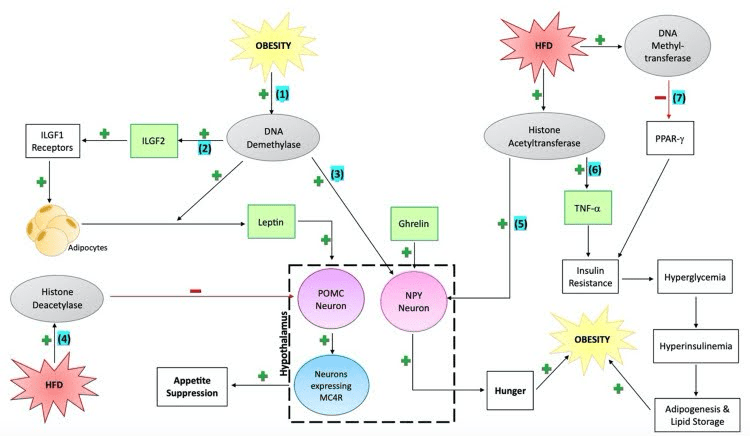
Figure 1. Seven epigenetic mechanisms of obesity (Smith et al., 2023). The figure demonstrates the connection between a high-fat diet and obesity through epigenetic modifications. Obesity may cause increasing hunger and overeating due to changes in gene expression for leptin, NPY, and POMC. Additionally, increased fat storage and insulin resistance may be caused due to changes in gene expression for PPAR-gamma and TNF-alpha.
Diabetes
Diabetes is a chronic metabolic disease distinguished by hyperglycemia, usually resulting from a malfunction in insulin production or action; it is mostly categorized in two types. Type 1 diabetes is characterized by a complete lack of insulin secretion, often linked to autoimmune processes and genetic markers. Conversely, type 2 diabetes, which is more common, results from a combination of insulin resistance and insufficient insulin secretion. Individuals with type 2 diabetes may experience hyperglycemia for an extended period without noticeable symptoms, making early detection through glucose testing essential (American Diabetes Association, 2010).
Even short-term changes to a diet may induce significant epigenetic modifications, therefore the correlation between epigenetics and metabolic disorders provides crucial insight to potential treatment and prevention of chronic metabolic disorders like diabetes (Heerboth et al., 2014). Both human studies and those in experimental animal models have shown that maternal obesity can programme increased risk of offspring developing obesity and adipose tissue dysfunction; type 2 diabetes with peripheral insulin resistance and β-cell dysfunction; CVD with impaired cardiac structure and function and hypertension via impaired vascular and kidney function (Inzani and Ozanne, 2022).
Early research on the relationship between type 2 diabetes (T2D) and epigenetics was heavily supported by the findings of altered methylation patterns in individuals with T2D (Ling and Rönn, 2019). Additionally, hypomethylation of the mitogen-activated protein kinase 4 (MAPK4) gene was found. These changes in methylation patterns were positively correlated with increased body weight, hyperglycemia, and insulin resistance in offspring. Offspring of mothers who were fed a high-fat diet were found to have hyperinsulinemia, hyperglycemia, and increased LPL and leptin gene expressions (Campión et al., 2023; Smith et al., 2023).
Cancer
In the United States alone, cancer ranks as the second most common cause of mortality across all age groups, with the exception of those under the age of 85, where it emerges as the primary cause of death. Colorectal cancer is now the leading cause of cancer death in men under 50 and the second-leading cause in women, despite ranking fourth in 1998 (Siegel et al., 2024). In recent months, discussions and posts raising awareness about colorectal cancer have gone viral on social media due to the spike in diagnoses.
Cancer is defined as a genetic disease in which cells divide uncontrollably. However, it is not a singular disease but rather a group of diseases, each exhibiting distinct characteristics. Cancer can arise in any part of the body and has the potential to metastasize, or spread to other organs and tissues, impairing their function (National Institutes of Health – U.S., 2007). Normally, aging or damaged cells are naturally replaced by new ones through cell division, but if there is a disturbance in this process, it may lead to the unregulated proliferation of abnormal or damaged cells (National Cancer Institute, 2021). Consequently, this process can lead to the development of masses of tissue referred to as tumors. Tumors are generally life-threatening when they disturb important organ functions, especially when they have spread to multiple organs (National Cancer Institute, 2021). It is important to note that not all types of cancer result in the formation of solid tumors, such as leukemia, lymphoma, and myeloma.
It is widely acknowledged that genetics play a significant role in cancer development. Genetic changes have the potential to enable precancerous cells to acquire characteristic features that collectively contribute to the development of malignant cancer cells. There are two groups of genes that work together to control the cell cycle (cell division and enlargement): proto-oncogenes, which stimulate cell division and proliferation, and tumor suppressor genes, which inhibit cell proliferation. When mutations occur in proto-oncogenes, they may overproduce cancerous cells. In tumor suppressor genes, the inhibitory messages may become blocked in cancer cells, allowing them to divide uncontrollably (Chakrabarti and Chattopadhyay, 2024).
Mutated proto-oncogenes, known as oncogenes, possess the capacity to induce cancer and may be affected by epigenetic alterations (Genome.gov, 2024). Epigenetics is something that heavily influences the result and progression of cancer treatment (oncological outcomes) (Chakrabarti and Chattopadhyay, 2024). Epigenetic alterations, encompassing modifications in histones and DNA methylation at specific CpG sites, are essential in carcinogenesis. Mutations activate oncogenes like RAS and SRC, while tumor suppressor genes, including p21, p16, p27, RARβ2, and ARHI, are often silenced via CpG island methylation in their promoters. DNMT1, upregulated in cancer cells, catalyzes methylation, leading to recruitment of methyl CpG-binding proteins and HDACs, hindering RNA polII-mediated transcription and promoting closed chromatin formation (National Institutes of Health – U.S., 2007). Unlike irreversible genetic mutations, epigenetic changes are reversible, making them attractive targets for therapy. Targeting silenced tumor suppressor genes with epigenetic drugs could potentially sensitize cancer cells to cytotoxic treatment, enhancing the efficacy of anticancer therapy (National Institutes of Health – U.S., 2007). With this discovery, cancer is now known to require both genetic alterations and abnormalities in the epigenome to form.
In many disease processes, such as cancer, gene promoter CpG islands acquire abnormal hypermethylation by altering DNMT expression and activity, which results in heritable transcriptional gene silencing. DNA methylation may impact the transcription of genes in two major ways. First, the methylation of DNA prevents binding of sequence specific transcriptional factors (e.g., AP-2, E2F, c-Myc) that require the presence of unmethylated CpG within the binding sites (Tate and Bird, 1993). Second, and likely more important, is that methylated DNA may be bound by proteins known as methyl-CpG-binding domain (MBD) proteins. MBD proteins then recruit additional proteins, such as histone deacetylases and other chromatin-remodeling proteins, that can modify acetylation and methylation status of histones to the locus thereby inhibiting transcriptional access to the chromatin (Meeran et al., 2010).
Epigenetic regulation has attracted considerable interest as a molecular target for cancer prevention and therapy, as well as a target of bioactive food components. Many bioactive dietary components have shown promising results in direct or indirect inhibition of DNMT activity in cancer prevention and therapy. For example, (−)-epigallocatechin-3-gallate (EGCG), a major component of green tea, is known to complex with the DNMTs which reduces methylating activity in cancer cells leading to cancer prevention or therapy through epigenetic mechanisms (Fang et al., 2003; Mittal et al., 2003; Tsao et al. 2009; Meeran et al., 2010).
Early developmental disruptions are associated with the onset of breast cancer in adulthood. Developmental Origins of Health and Disease (DOHaD) theorized that in certain stages of early development, epigenetic plasticity reaches its peak, elevating the risk of cancer development due to environmental exposures. In the case of breast cancer, the majority of evidence regarding its inheritance primarily stems from maternal in-utero exposures during pregnancy. It was established in the 1990s that exposure to elevated estrogen levels in utero could lead to breast cancer. Diet is a factor that can significantly influence estrogen levels; therefore, modifying maternal diet during pregnancy to reduce spiked estrogen levels could be one way to reduce the chances of breast cancer (daCruz et al., 2020).
Cardiovascular disease/conditions
Epigenetics is closely related to cardiovascular diseases. Cardiovascular diseases (CVD) include coronary heart disease, hypertension, heart failure, and vascular calcification, among other conditions. Genome-wide linkage and association analyses and candidate gene approaches illustrate the multigenic complexity of CVD. Several epigenetic mechanisms, such as DNA methylation, histone modification, and noncoding RNA, are of importance for CVD development and regression. Targeting epigenetic key enzymes, especially the DNA methyltransferases, histone methyltransferases, histone acetylases, histone deacetylases and their regulated target genes, could represent an attractive new route for the diagnosis and treatment of CVD. Epigenetics mainly regulates CVD-related genes function and expression level through DNA methylation, histone modification, and noncoding RNA regulation; thus, affecting CVD progression. Because of the reversibility of epigenetic modifications, genes and proteins that control these changes have become new targets for CVD treatment (Shi et al., 2022).
Resveratrol has a protective effect on atherosclerosis, coronary heart disease, ischemia–reperfusion injury, heart failure, hypertension, and pulmonary hypertension by regulating histone modification-related factors. Trichostatin A and P300 HAT inhibitor curcumin can improve the progression of atherosclerosis, coronary heart disease and heart failure. Histone modification inhibitors resveratrol, Trichostatin A and Curcumin are expected to be widely used in the treatment of various CVDs through further clinical studies (Shi et al., 2022).
A surplus of macronutrients, such as in a high fat diet, or deficiencies of specific nutrients, such as folate and other B-vitamins, can affect the activity of DNA methyltransferases and histone modifying enzymes, affecting fetal growth, glucose/lipid metabolism, oxidative stress, inflammation and atherosclerosis. Bioactive compounds, such as polyphenols (resveratrol, curcumin) or epigallocatechin, may activate deacetylases SIRTs, histone deacetylases or acetyltransferases and, in turn, the response of inflammatory mediators like folic acid, B-vitamins, and other methyl donors (Kalea et al., 2018). The epigenetic effects of methyl-donor nutrients on vascular aging and cardiometabolic risk are better understood. Dietary intake of methyl-group donors and co-factors during pregnancy has been reported to affect fetal growth and development, thus establishing a major link between early environmental exposure and chronic disease development in the offspring later in life (Kalea et al., 2018; Franke et al., 2018; van Dijk et al., 2016).
Epigenetic alterations help elucidate the mechanisms by which diet and nutrients may affect gene expression and regulation. Dysregulation of the epigenome, including changes in DNA methylation and histone acetylation, plays a major role in CVD. A surplus of macronutrients (fatty acids and carbohydrates) or nutrient deficiencies (folate and other B-vitamins), as well as bioactive compounds, such as polyphenols (resveratrol, curcumin) or epigallocatechin, may affect the expression levels of methylation and acetylation enzymes, which affect the expression of genes that are linked to vascular dysfunction. Identification of the causal directions of the relationships between dietary patterns, epigenetic changes, telomere length and disease risk will facilitate the development of treatment and intervention measures (Kalea et al., 2018).
Other conditions
Maternal overnutrition during sensitive periods of early development increases the risk for obesity and neuropsychiatric disorders later in life. Developmental Origins of Health and Disease (DOHaD) hypothesize that in certain stages of early development, epigenetic plasticity reaches its peak, elevating the risk of cancer development due to environmental exposures. However, it still remains unclear during which phases of early development the offspring is most vulnerable. The maternal high-fat diet (MHFD) was investigated to determine the effects of its application at different stages of pre- or postnatal development in order to characterize the behavioral, neurochemical and metabolic phenotypes. Offspring exposed to MHFD during lactation display the metabolic syndrome and schizophrenia-like phenotype. Lactational MHFD exposure induces schizophrenia-like behaviors in the offspring, for instance, Prepulse Inhibition (PPI) impairment and disruption of Latent Inhibition (LI). PPI refers to sensory motor gating deficits and LI disruption reflects attention deficits. Both have been commonly reported in schizophrenic patients. In previous studies, it was observed that time specific MHFD exposure is associated with profound metabolic alterations, enhanced addictive-like and schizophrenia-relevant behaviors in the offspring later in life. Postmortem neurochemical analysis further showed that the metabolic and behavioral abnormalities are accompanied with sustained alterations in dopamine levels in the reward related brain regions (Sarker et al., 2019).
Beneficial nutrients/compounds in foods
The bioactive components of dietary phytochemicals most often shown to be effective against cancer are tea polyphenols, genistein, curcumin, resveratrol, sulforaphane, isothiocyanates, silymarin, diallyl sulfide, lycopene, rosmarinic acid, apigenin, and gingerol. These bioactive components have shown great potential in preventing cancer through modifying genetic and epigenetic targets (Meeran et al., 2010).
Although several dietary agents or nutrients regulate different molecular targets in various cancers, Table 1 summarizes the role of some common bioactive dietary agents and their epigenetic targets on various cancers. The agents listed and their dietary sources include tea polyphenol–catechins (green tea), curcumin (turmeric), genistein (soybean), resveratrol (grapes), SFN (cruciferous vegetables), and other bioactive components such as apigenin (parsley), baicalein (Indian trumpet), cyanidins (grapes), isothiocyanate (cruciferous vegetables), rosmarinic acid (rosemary), and silymarin (milk thistle) (Meeran et al., 2010).
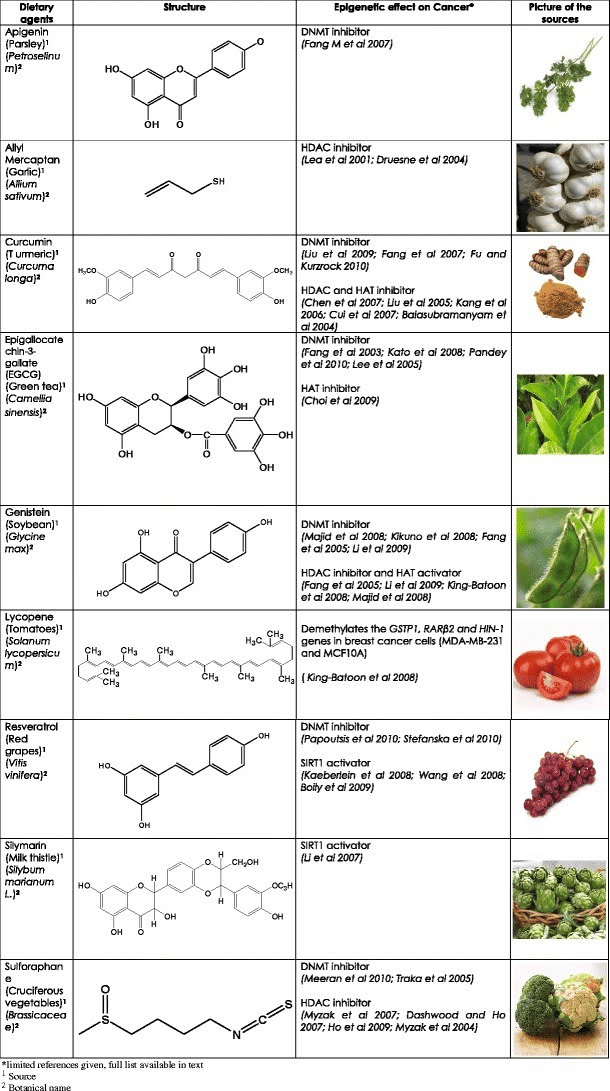
Table 1. Bioactive dietary compounds — source, molecular structure, and epigenetic targets (Meereen et al., 2010).
What steps can be taken?
Preconception conditions
Due to the inheritability of gene expression or silencing, the preconception of obesity of the parents can pass these epigenetic conditions to the children. Both maternal and paternal obesity prior to conception can contribute to these conditions leading to obesity in the offspring. Awareness of the epigenetic effects of the preconception nutritional intake is critical to providing the offspring the best opportunity to avoid epigenetic pitfalls that can last into adulthood and to subsequent generations.
Avoiding obvious factors
Just as nutrition can affect the inheritance of adverse epigenetic gene expression or silencing, other health factors, such as addictive agents (i.e. smoking and alcohol), prescriptive medicine, environmental pollutants, and stress have been found to affect gene expression that can be inherited by offspring. There are three categorical stages of life history when epigenetics are registered: ancestral (including parents), prenatal, and postnatal stages. The prenatal epigenetic registration is the most significant influence on the formation of the nervous system during development. Environmental inputs prior to birth are reported to impose epigenetic entries, many of which are carried throughout the life of somatic cells. Avoiding such factors limits the accumulation of epigenetic marks adversely affecting offspring health (Lo and Zhou, 2014). In the case of diseases like cancer, it is best to avoid them.
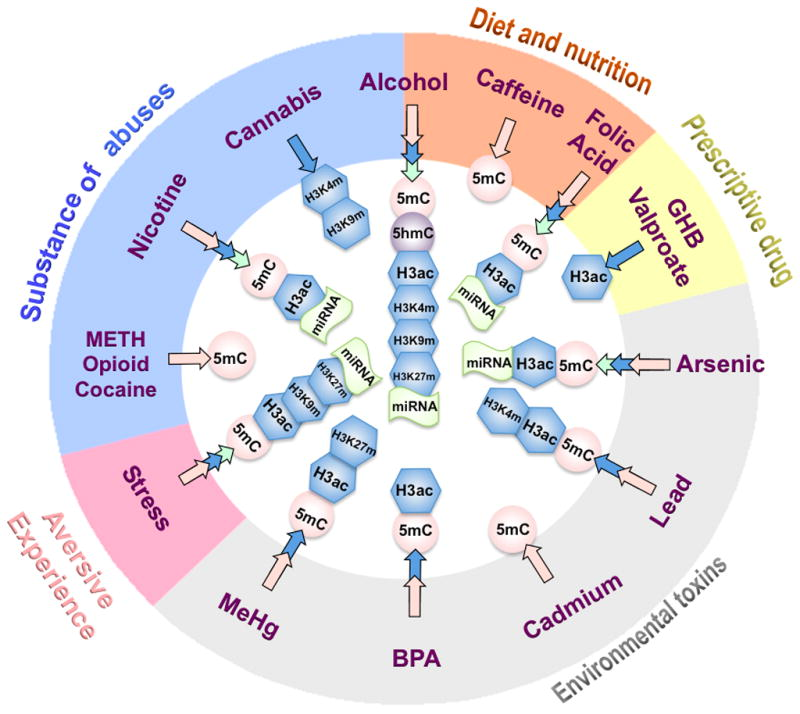
Figure 2: Environmental Factors Having Adverse Epigenetic Effects (Lo and Zhou, 2014).
Nutritional strategy for maternity
A recent study by Mahmassani et al. (2022) showed higher maternal dietary intake quality during pregnancy was associated with better visual-spatial skills, as well as cognitive scores in offspring at early childhood (Crovetto et al., 2023). In addition, there are also literature reports of positive associations with higher intelligence and executive functions at mid-childhood (Zupo et al., 2023; Na et al., 2023).
Developmental plasticity in mind
As an example, neonatal rats exposed to a low (10%), medium (18%) or high (36%) protein diet during gestation and lactation have a greater survival rate when their post-weaning diet matches the diet consumed by their respective dam (mother) during gestation and lactation (Sasaki et al., 1982). Thus, developmental plasticity allows the organism to thrive in the anticipated environment in which the fetus will emerge (Bateson et al., 2001).
More research needed
To determine common epigenetic pathways that lead from multiple environmental factors to the same results (i.e., many paths to obesity), further research is needed to determine underlying patterns and trends. The examination of cause and effect and the correlation of underlying mechanisms for the gamut of environmental factors, which lead to epigenetic marks, is necessary to develop strategies for preventing, treating, or reversing the negative epigenetic consequences.
Conclusion
Awareness of epigenetic connections to many public health issues and addressing the established triggers will improve public health. The importance of maternal health during the gestational period has always been emphasized for the well-being of the mother and for mitigating the risks involved in genetic disorders and diseases that have a clearly established cause-effect, such as alcohol fetal syndrome. However, healthcare providers may also consider equally emphasizing the importance of maternal health in terms of avoiding more insidious outcomes of an unfavorable maternal environment, such as fetal and childhood obesity that can persist into adult life. By making positive changes in quantity and quality of diet, avoiding epigenetically harmful substances, and including beneficial dietary components at the maternal level over the course of generations, it may be possible that the prevalence of obesity, as well as diabetes and cancer, can be greatly reduced.
Bibliography
Al-hussaniy, H. akeel, Altalebi, R.R., Tylor, F.M., Alwash, A.H., Naji, M.A. and Kadhim, Z.S. (2022). Leptin Hormone: In Brief. Medical and Pharmaceutical Journal, 1(1), pp.1–3. doi:https://doi.org/10.55940/medphar20221.
American Diabetes Association (2010). Diagnosis and classification of diabetes mellitus. Diabetes Care, [online] 33(Supplement_1), pp.S62–S69. doi: https://doi.org/10.2337/dc10-s062.
Ando, C., Ma, S., Miyoshi, M., Furukawa, K., Li, X., Jia, H. and Kato, H. (2023). Postnatal nutrition environment reprograms renal DNA methylation patterns in offspring of maternal protein-restricted stroke-prone spontaneously hypertensive rats. Frontiers in Nutrition, 10. doi: https://doi.org/10.3389/fnut.2023.1134955.
Bateson, P. (2001). Fetal experience and good adult designa. International Journal of Epidemiology, 30(5), pp.928–934. doi: https://doi.org/10.1093/ije/30.5.928.
Bateson, P., Barker, D., Clutton-Brock, T., Deb, D., D’Udine, B., Foley, R.A., Gluckman, P., Godfrey, K., Kirkwood, T., Lahr, M.M., McNamara, J., Metcalfe, N.B., Monaghan, P., Spencer, H.G. and Sultan, S.E. (2004). Developmental plasticity and human health. Nature, 430(6998), pp.419–21. doi: https://doi.org/10.1038/nature02725.
Begüm Harmancıoğlu and Seray Kabaran (2023). Maternal high fat diets: impacts on offspring obesity and epigenetic hypothalamic programming. Frontiers in Genetics, 14. doi: https://doi.org/10.3389/fgene.2023.1158089.
Campión, J., Milagro, F. and Martínez, J.A. (2010). Epigenetics and Obesity. Progress in Molecular Biology and Translational Science, pp.291–347. doi: https://doi.org/10.1016/b978-0-12-375003-7.00011-x.
Chakrabarti, S.K. and Chattopadhyay, D. (2024). Expanding Role of Epigenetics in Human Health and Disease. Exploratory Research and Hypothesis in Medicine. doi: https://doi.org/10.14218/ERHM.2023.00086.
Crovetto, F., A. Nakaki, A. Arranz, Borràs, R., Kilian Vellvé, Paules, C., Boutet, M.L., Castro-Barquero, S., Freitas, T., Casas, R., Andrés Martín-Asuero, Teresa Oller Guzmán, Morilla, I., Martinez-Aran, A., Camacho, A., Pasqual, M., Montserrat Izquierdo Renau, Pozo, O.J., Gomez-Gomez, A. and Estruch, R. (2023). Effect of a Mediterranean Diet or Mindfulness-Based Stress Reduction During Pregnancy on Child Neurodevelopment. JAMA network open, 6(8), pp.e2330255–e2330255. doi: https://doi.org/10.1001/jamanetworkopen.2023.30255.
da Cruz, R. S., Chen, E., Smith, M., Bates, J., & de Assis, S. (2020). Diet and Transgenerational Epigenetic Inheritance of Breast Cancer: The Role of the Paternal Germline. Frontiers in nutrition, 7, 93. doi: https://doi.org/10.3389/fnut.2020.00093.
Dobbs, R., Sawers, C., Thompson, F., Manyika, J., Woetzel, J., Child, P., McKenna, S. and Spatharou, A. (2014). Overcoming obesity: An initial economic analysis. Available at: https://www.mckinsey.com/~/media/mckinsey/business%20functions/economic%20studies%20temp/our%20insights/how%20the%20world%20could%20better%20fight%20obesity/mgi_overcoming_obesity_executive_summary.pdf.
Fang, M.Z., Wang, Y., Ai, N., Hou, Z., Sun, Y., Lu, H., Welsh, W. and Yang, C.S. (2003). Tea polyphenol (-)-epigallocatechin-3-gallate inhibits DNA methyltransferase and reactivates methylation-silenced genes in cancer cell lines. Cancer Research, [online] 63(22), pp.7563–7570. Available at: https://pubmed.ncbi.nlm.nih.gov/14633667/.
Feehley, T., O’Donnell, C.W., Mendlein, J., Karande, M. and McCauley, T. (2023). Drugging the epigenome in the age of precision medicine. Clinical Epigenetics, 15(1). doi: https://doi.org/10.1186/s13148-022-01419-z.
Franke, K., Gaser, C., Roseboom, T.J., Schwab, M. and de Rooij, S.R. (2018). Premature brain aging in humans exposed to maternal nutrient restriction during early gestation. NeuroImage, 173, pp.460–471. doi: https://doi.org/10.1016/j.neuroimage.2017.10.047.
Genome.gov. (n.d.) (2024). Oncogene. National Human Genome Research Institute. Available at: https://www.genome.gov/genetics-glossary/Oncogene#:~:text=An%20oncogene%20is%20a%20mutated.
Heerboth, S., Lapinska, K., Snyder, N., Leary, M., Rollinson, S. and Sarkar, S. (2014). Use of Epigenetic Drugs in Disease: An Overview. Genetics & Epigenetics, [online] 6, p.GEG.S12270. doi: https://doi.org/10.4137/geg.s12270.
Inzani, I. and Ozanne, S.E. (2022). Programming by maternal obesity: a pathway to poor cardiometabolic health in the offspring. The Proceedings of the Nutrition Society, [online] 81(3), pp.227–242. doi: https://doi.org/10.1017/S0029665122001914.
Jaenisch, R. and Bird, A. (2003). Epigenetic regulation of gene expression: how the genome integrates intrinsic and environmental signals. Nature Genetics, 33(S3), pp.245–254. doi: https://doi.org/10.1038/ng1089.
Kalea, A.Z., Drosatos, K. and Buxton, J.L. (2018). Nutriepigenetics and cardiovascular disease. Current opinion in clinical nutrition and metabolic care, 21(4), pp.252–259. doi: https://doi.org/10.1097/MCO.0000000000000477.
Ling, C. and Rönn, T. (2019). Epigenetics in Human Obesity and Type 2 Diabetes. Cell Metabolism, 29(5), pp.1028–1044. doi: https://doi.org/10.1016/j.cmet.2019.03.009.
Lo, C.-L. and Zhou, F.C. (2014). Environmental alterations of epigenetics prior to the birth. International Review of Neurobiology, [online] 115, pp.1–49. doi: https://doi.org/10.1016/B978-0-12-801311-3.00001-9.
Mahmassani, H.A., Switkowski, K.M., Scott, T.M., Johnson, E.J., Rifas-Shiman, S.L., Oken, E. and Jacques, P.F. (2021). Maternal diet quality during pregnancy and child cognition and behavior in a US cohort. The American Journal of Clinical Nutrition. doi: https://doi.org/10.1093/ajcn/nqab325.
Mahmoud, A.M. (2022). An Overview of Epigenetics in Obesity: The Role of Lifestyle and Therapeutic Interventions. International Journal of Molecular Sciences, [online] 23(3), p.1341. doi: https://doi.org/10.3390/ijms23031341.
Meeran, S.M., Ahmed, A. and Tollefsbol, T.O. (2010). Epigenetic targets of bioactive dietary components for cancer prevention and therapy. Clinical Epigenetics, 1(3-4), pp.101–116. doi: https://doi.org/10.1007/s13148-010-0011-5.
Mittal, A., Chandrika Piyathilake, Hara, Y. and Katiyar, S.K. (2003). Exceptionally High Protection of Photocarcinogenesis by Topical Application of (-)-Epi gal locatechin-3-Gal late in Hydrophilic Cream in SKH-1 Hairless Mouse Model: Relationship to Inhibition of UVB-Induced Global DNA Hypomethylation. Neoplasia, 5(6), pp.555–565. doi: https://doi.org/10.1016/s1476-5586(03)80039-8.
Moosavi, A. and Motevalizadeh Ardekani, A. (2016). Role of Epigenetics in Biology and Human Diseases. Iranian biomedical journal, [online] 20(5), pp.246–58. doi: https://doi.org/10.22045/ibj.2016.01
Morrison, J. and Regnault, T. (2016). Nutrition in Pregnancy: Optimising Maternal Diet and Fetal Adaptations to Altered Nutrient Supply. Nutrients, [online] 8(6), p.342. doi: https://doi.org/10.3390/nu8060342.
Na, X., Glasier, C.M., Andres, A. and Ou, X. (2023). Maternal Diet Quality during Pregnancy Is Associated with Neonatal Brain White Matter Development. Nutrients, [online] 15(24), p.5114. doi: https://doi.org/10.3390/nu15245114.
National Cancer Institute (2021). What Is Cancer? – National Cancer Institute. Available at: https://www.cancer.gov/about-cancer/understanding/what-is-cancer.
National Institutes of Health (US); Biological Sciences Curriculum Study. NIH Curriculum Supplement Series [Internet]. Bethesda (MD): National Institutes of Health (US); 2007. Understanding Cancer. Available at: https://www.ncbi.nlm.nih.gov/books/NBK20362/.
News-Medical. (2021). Eating low-quality diet during pregnancy is linked to late-childhood obesity. Available at: https://www.news-medical.net/news/20210221/Eating-low-quality-diet-during-pregnancy-is-linked-to-late-childhood-obesity.aspx [Accessed 14 Feb. 2024].
Obesity Prevention Source. (2012). Prenatal and Early Life Influences. Available at: https://www.hsph.harvard.edu/obesity-prevention-source/obesity-causes/prenatal-postnatal-obesity/.
Parlee, S.D. and MacDougald, O.A. (2014). Maternal nutrition and risk of obesity in offspring: The Trojan horse of developmental plasticity. Biochimica et Biophysica Acta (BBA) – Molecular Basis of Disease, [online] 1842(3), pp.495–506. doi: https://doi.org/10.1016/j.bbadis.2013.07.007.
Peixoto, P., Cartron, P.-F., Serandour, A.A. and Hervouet, E. (2020). From 1957 to Nowadays: A Brief History of Epigenetics. International Journal of Molecular Sciences, 21(20), p.7571. doi: https://doi.org/10.3390/ijms21207571.
Perla Pizzi Argentato, Augusto, J., Naiara Naiana Dejani, Patrícia Yury Nakandakare, Teles, S., Rodrigues, P., Paula, M., Liania Alves Luzia, Ester Silveira Ramos and Patricia Helen Rondó (2023). The relationship between obesity-related H19DMR methylation and H19 and IGF2 gene expression on offspring growth and body composition. Frontiers in Nutrition, 10. doi: https://doi.org/10.3389/fnut.2023.1170411.
Rasool, M., Malik, A., Naseer, M.I., Manan, A., Ansari, S.A., Begum, I., Qazi, M.H., Pushparaj, P.N., Abuzenadah, A.M., Al-Qahtani, M.H., Kamal, M.A. and Gan, S.H. (2015). The role of epigenetics in personalized medicine: challenges and opportunities. BMC Medical Genomics, 8(S1). doi: https://doi.org/10.1186/1755-8794-8-s1-s5.
Sarker, G., Litwan, K., Kastli, R. and Peleg-Raibstein, D. (2019). Maternal overnutrition during critical developmental periods leads to different health adversities in the offspring: relevance of obesity, addiction and schizophrenia. Scientific Reports, 9(1). doi: https://doi.org/10.1038/s41598-019-53652-x.
Sasaki, A., Nakagawa, I. and Masatoshi Kajimoto (1982). Effect of protein nutrition throughout gestation and lactation on growth, morbidity and life span of rat progeny. Journal of Nutritional Science and Vitaminology, 28(5), pp.543–555. doi: https://doi.org/10.3177/jnsv.28.543.
Shi, Y., Zhang, H., Huang, S., Yin, L., Wang, F., Luo, P. and Huang, H. (2022). Epigenetic regulation in cardiovascular disease: mechanisms and advances in clinical trials. Signal Transduction and Targeted Therapy, 7(1). doi: https://doi.org/10.1038/s41392-022-01055-2.
Siegel, R.L., Giaquinto, A.N. and Jemal, A. (2024). Cancer statistics, 2024. CA: A Cancer Journal for Clinicians, 74(1). doi: https://doi.org/10.3322/caac.21820.
Smith, E.N.L., Chandanathil, M., Millis, R.M., Smith, E.N., Chandanathil, M. and Millis, R.M. (2023). Epigenetic Mechanisms in Obesity: Broadening Our Understanding of the Disease. Cureus, [online] 15(10). doi: https://doi.org/10.7759/cureus.47875.
Tate, P.H. and Bird, A.P. (1993). Effects of DNA methylation on DNA-binding proteins and gene expression. Current Opinion in Genetics & Development, 3(2), pp.226–231. doi: https://doi.org/10.1016/0959-437x(93)90027-m.
Tsao, A.S., Liu, D., Martin, J., Tang, X., Lee, J.J., El-Naggar, A.K., Wistuba, I., Culotta, K.S., Mao, L., Gillenwater, A., Sagesaka, Y.M., Hong, W.K. and Papadimitrakopoulou, V. (2009). Phase II Randomized, Placebo-Controlled Trial of Green Tea Extract in Patients with High-Risk Oral Premalignant Lesions. Cancer Prevention Research, 2(11), pp.931–941. doi: https://doi.org/10.1158/1940-6207.capr-09-0121.
Tyagi, S., Raghvendra and Tyagi, V. (2010). POTENTIAL MEDICAL APPLICATIONS OF EPIGENETICS: AN OVERVIEW. International Journal of Pharmaceutical Sciences Review and Research, [online] 3(1). Available at: https://www.globalresearchonline.net/journalcontents/volume3issue1/Article%20029.pdf [Accessed 14 Feb. 2024].
van Dijk, S.J., Jing Zhou, Peters, T.J., Buckley, M., Sutcliffe, B., Oytam, Y., Gibson, R.A., McPhee, A., Yelland, L.N., Makrides, M., Molloy, P.L. and Muhlhausler, B.S. (2016). Effect of prenatal DHA supplementation on the infant epigenome: results from a randomized controlled trial. Clinical Epigenetics, [online] 8, pp.1–13. doi: https://doi.org/10.1186/s13148-016-0281-7.
Waddington, C.H. (1942). The Epigenotype. International Journal of Epidemiology, 41(1), pp.10–13. doi: https://doi.org/10.1093/ije/dyr184.
Waterland, R.A. and Jirtle, R.L. (2004). Early nutrition, epigenetic changes at transposons and imprinted genes, and enhanced susceptibility to adult chronic diseases. Nutrition, 20(1), pp.63–68. doi: https://doi.org/10.1016/j.nut.2003.09.011.
West-Eberhard, M.J. (2003). Developmental Plasticity and Evolution. [online] Oxford University Press. doi: https://doi.org/10.1093/oso/9780195122343.001.0001.
World Health Organization (2021). Obesity and overweight. [online] World Health Organization. Available at: https://www.who.int/news-room/fact-sheets/detail/obesity-and-overweight.
Wu, G., Bazer, F.W., Cudd, T.A., Meininger, C.J. and Spencer, T.E. (2004). Maternal Nutrition and Fetal Development. The Journal of Nutrition, [online] 134(9), pp.2169–2172. doi: https://doi.org/10.1093/jn/134.9.2169.
Zoghbi, H.Y. and Beaudet, A.L. (2016). Epigenetics and Human Disease. Cold Spring Harbor Perspectives in Biology, [online] 8(2), p.a019497. doi: https://doi.org/10.1101/cshperspect.a019497.
Zupo, R., Castellana, F., Boero, G., Matera, E., Colacicco, G., Piscitelli, P., Clodoveo, M.L., Rondanelli, M., Panza, F., Lozupone, M. and Sardone, R. (2023). Processed foods and diet quality in pregnancy may affect child neurodevelopmental disorders: a narrative review. Nutritional Neuroscience, pp.1–21. doi: https://doi.org/10.1080/1028415x.2023.2197709.