Supervised by: Amanda Liu, BEng, MSc. Amanda spent her undergraduate years studying Biochemical Engineering at UCL (University College London), where she was awarded First Class Honours. She then completed her Master’s degree in Clinical and Therapeutic Neuroscience at the University of Oxford. She is currently studying Medicine (Graduate Entry) at the University of Cambridge.
Abstract
Stem cell therapy has long been a debatable treatment for patients, especially those diagnosed with neurological diseases such as Parkinson’s. In Parkinson’s disease, the loss and damage of dopaminergic neurons cause over 10 million patients to suffer from daily tremors, stiffness, and rigid movements. While conventional treatments such as L-DOPA and deep brain stimulation have provided patients with partial symptomatic relief, fatigability has posed significant limits in further treatment of the disease. This is where stem cell therapy displays significant potential, considering it is a new treatment that has been introduced in the medical field. This research analyzes several experiments observing the efficacy and safety of stem cell therapy on animal subjects and human fibroblasts with Parkinson’s disease. This paper also considers stem cell therapy’s ethical and financial implications of stem cell therapy, alongside the projected results, including increased survival rates and significant symptomatic relief of subjects. The review suggests umbilical cord blood stem cells and induced pluripotent stem cells as the two most viable types of stem cells for treating Parkinson’s Disease.
Keywords
Totipotent, Pluripotent, Multipotent, Proliferative
Parkinson’s Disease (PD), Dopaminergic Neurons (DN), L-DOPA, UCMS, Stem Cells (SCs), Induced Pluripotent Stem Cells (iPSCs), Embryonic Stem Cells (EPSCs), Deep brain stimulation (DBS)
Introduction
Stem Cells
Stem cells are unspecialized cells that can regenerate and proliferate into specialized cells via mitosis. They have unique self-renewal properties, which means they can divide and renew themselves for indefinite periods of time. These qualities of stem cells are highly beneficial, as they can replace or optimize the functioning of diseased or degenerating tissue. They can be cultured in labs to give rise to new nerve cells, heart cells, gut cells, etc. Their potency determines the ability of stem cells to transform into specialized cells; they can be categorized as totipotent, pluripotent, or multipotent.
Totipotent cells are found in the initial stages of zygote formation. They can regenerate into any type of cell in the body, including extraembryonic cells found in the placenta and umbilical cord. Pluripotent cells are exclusively found inside the developing blastocyst and can develop into multiple specialized cell types but are limited in numbers.
These are the main types of stem cells: Embryonic stem cells (ESCs), Tissue-specific stem cells (TSCs), and Induced Pluripotent stem cells (iPSCs).
The presence of stem cells is particularly evident in human embryos. As pluripotent cells, Embryonic stem cells (ESCs) develop into cells from any of the three germ layers. A collection of cells in the embryo is known as a germ layer. As the embryo grows, these layers interact and develop into the organs and tissues of the organism. The three layers are the endoderm (inner layer), the mesoderm (middle layer), and the ectoderm (outer layer). They are all collectively known as the germ layer. The first multipotent stem cells are found in these layers during the formation of a zygote, where they allow each germ layer to develop into specific types of tissue. This process will eventually lead up to the formation of an organism.
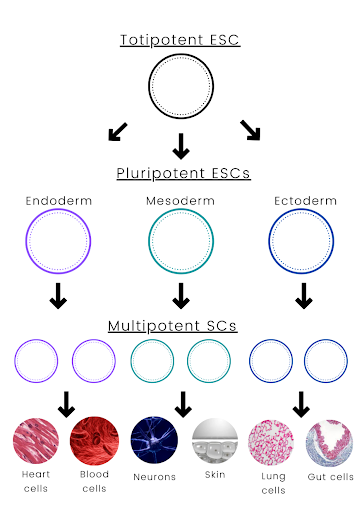
Figure 1: A flowchart representation of how stem cells become specialized into specific tissues.
ESCs can be extracted from a blastocyst’s inner cell mass (ICM) of a blastocyst. They can be cultured in laboratories, given their highly proliferative properties. However, extraction of ESCs results in the destruction of the human embryo, which has ethical concerns. Although the process of using human ESCs has only been recently approved for research, their effectiveness and capabilities provide promising results for the future of regenerative medicine.
Tissue-specific stem cells (TSCs), also known as adult or somatic stem cells, can only replicate the cells for the tissue or organ they live in. They are limited in producing a variety of cells when compared to ESCs. Their primary function is to create new cells that replace the damaged ones. Adult bone marrow is a site of TSCs, where the bone marrow can generate platelets, red blood cells, and white blood cells. Hematopoietic stem cells present in bone marrow can only produce these cells specifically. This attribute is why they are called adult stem cells, as these stem cells function throughout the life of an organism. They are not as effective when cultured in labs in comparison to ESCs.
Induced pluripotent stem cells (iPSCs) are generated from adult somatic cells that have been genetically reprogrammed through gene and protein expression to form a pluripotent state like that of ESCs. This allows for the development of an infinite source of any type of human cell required for therapeutic applications. IPSCs can then differentiate into any cell in the body, regardless of the original tissue from which they were derived.
Parkinson’s Disease
Parkinson’s disease (PD) is a neurological condition characterized by resting tremors, stiffness, slow movement, and postural instability. These symptoms are produced by neuron degeneration in the substantia nigra pars compacta (SNc), one of the basal ganglia: a set of brain regions essential for coordinating purposeful movement. This circuit depends on dopamine, a chemical messenger (or neurotransmitter) released by the SNc neurons. Reduced dopamine leads to irregular circuit activity and motor symptoms. As PD advances, these neurons are damaged even further.
PD is the second most prevalent neurodegenerative illness after Alzheimer’s disease. The population prevalence of Parkinson’s disease rises from 1% at age 60 to 4% by age 80 (CDC, 2019). 10 million people worldwide are diagnosed with PD each year, and people younger than 50 diagnosed with PD are considered to have Young Onset Parkinson’s Disease (YOPD).
Tremors, stiffness, and trouble walking are early signs of Parkinson’s disease; cognitive deterioration is prevalent in later stages. PD prevalence rises with age and affects 1-2 per 1000 people at any given moment (Tysnes & Storstein, 2017).
The aetiology of Parkinson’s disease is characterized by the loss of nigrostriatal dopaminergic innervation (Kouli et al., 2018). Environmental and Genetic factors are also thought to play a role in increasing the risk of the disease.
The lack of dopamine in the substantia nigra generates abnormal brain activity, resulting in impaired mobility and other Parkinson’s disease symptoms. The clinical features include bradykinesia (slowed movement), tremors, impaired posture and loss of balance, changes in speech patterns, and many more.
The deterioration of substantial nigra neurons and the loss of dopaminergic projections result in the hyperactivity of indirect pathways in Parkinson’s disease, which results in greater inhibition of motor regions of the brain and diminished movements.
Levodopa
Levodopa (L-DOPA), the molecular precursor of dopamine, is commonly used to treat Parkinson’s disease. However, people in the advanced stages of the illness encounter “off” periods when this drug does not work effectively. L-DOPA therapy can also cause uncontrollable involuntary movement, a condition known as dyskinesia. More than 50% of PD patients treated with levodopa for more than 5 years develop levodopa-induced dyskinesia (LID) (Eusebi et al., 2018).
Deep Brain Stimulation
The human brain has billions of neurons that communicate with one another via electrical and chemical impulses. Several brain diseases, like Parkinson’s, can cause neurons in various sections of the brain to become less active. When this happens, those areas stop functioning correctly. This may cause disturbances in the abilities regulated by the damaged section of the brain. Deep brain stimulation (DBS) is one of the most performed surgical treatments for PD. A surgeon inserts thin metal wires into the brain, which emit electrical pulses to help regulate some motor symptoms.
DBS deploys an artificial electrical current to stimulate certain neurons, which can assist with the symptoms of PD and various brain diseases. DBS can provide symptomatic relief in the latter stages of PD and can also minimize the need for L-DOPA medication and exposure to its side effects.
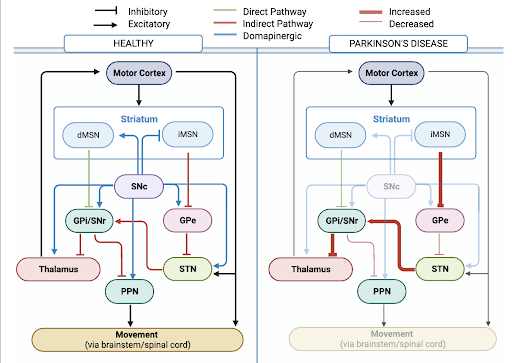
Figure 2: The diagram depicts the basal ganglia in both healthy and Parkinson’s disease conditions.
Hypothesis
Stem cell therapy with Induced Pluripotent stem cells will be the most effective treatment for Parkinson’s disease, as patients will have long-term benefits without harmful side effects caused by conventional medication.
Methods
As there are currently no cures for PD, pharmacotherapy for relieving motor symptoms focuses on restoring striatal dopamine through medications that include dopamine agonists, monoamine oxidase (MAO)-B inhibitors, L-DOPA plus carbidopa, and COMT (catechol-O-methyltransferase) inhibitors.
Considering that dopamine is produced through the conversion of L-tyrosine to L-DOPA by tyrosine hydroxylase (TH) and decarboxylation of L-DOPA by dopa decarboxylase, L-DOPA is a crucial element in dopamine replacement therapy. While these drugs may create partial symptomatic relief, their significant limitations challenge recovery from PD as the effects usually diminish over time. Patients often experience side effects such as hallucinations and cognitive impairment. Patients exhausted from such significant side effects may also be advised to undergo deep brain stimulation or surgery, defying the purpose of drug treatment therapy and making it less effective. This also largely increases the risks of treating the disease. As an alternative option, researchers believe that DA cell transplantation, more specifically, stem cell therapy, is the most promising. Clinical trials have revealed that transplanting midbrain Dopamine (DA) neurons into DA-depleted striatum allows for significant recovery of DA neurotransmission. The DA neurons are synthesized from human neural stem cells, mesenchymal stem cells, human ESCs, IPSCs and bone-marrow-derived stem cells (Liu, Cheung).
Here, the outcome of a clinical trial is considered positive when the transplanted cells survive/are constantly active, provide functional dopaminergic reinnervation of the striatum, and provide sufficient symptomatic relief. While the efficacy and safety of stem cell therapy in Parkinson’s Disease are yet to be confirmed, clinical trials have shown extensive progress and ever-growing potential in the treatment of the disease.
Injection of Mesenchymal Stem Cells Derived from the Human Umbilical Cord
Researchers have long suggested that blood-forming stem cells (hematopoietic stem cells [HSCs]) and mesenchymal stem cells (MSCs) in the human umbilical cord might have a positive effect on neurological diseases, especially PD (Weiss, Medicetty, et al.). In research conducted by UMD-New Jersey Medical School, 32 homozygous mice 6-12 weeks of age were obtained from Jackson Laboratory Bar Harbor, Maine, to observe the effects of human umbilical cord blood on the symptoms and death of PD mice. The mice were divided into three groups: 10 mice that received no treatment (control group), 10 mice that received 5.6*106 monocular bone marrow cells from congenic mice, and 12 mice that received 100-110*106 cord blood mononuclear cells across two doses (50-60*106), each injected 24 hours apart. The human umbilical cord blood samples were extracted from the placentas of healthy neonates, each into a 50 mL sterile polypropylene test tube that contained 5mL Citrate Phosphate Dextrose to serve as an anticoagulant. Before injection, the samples were kept at room temperature and transferred into a blood bank with a polyolefin blood collection back within 24 hours. The samples were kept at 4°C at the blood bank for 10 to 13 days. After 48-72 hours, donor specimens were combined based on their blood type (ABO). The mononuclear cells (MNC) were then separated from the rest of the cord blood by centrifugation for 30 minutes at 1700 RPMs for injection. After 200 days, 9 out of the 10 mice in the control group, 8 out of the 10 mice receiving congenic marrow, and 8 out of the 12 receiving cord blood died. Compared to the untreated controls, mice treated with congenic marrow or cord blood displayed higher survival rates.
In another research, umbilical cord matrix stem cells (UCMS) derived from the human umbilical cord Wharton’s Jelly were transplanted into Hemi parkinsonian rats to experiment with the therapeutic value of USMC cells. Here, brain lesions were created in the hemiparkinsonian rat model by a single stereotaxic injection of 6-hydroxydopamine (6-OHDA) – inducing rapid damage to dopaminergic neurons. After 4 weeks, approximately 1000 UCMS cells were injected into the striatum of 12 randomly assigned subjects. At 12 weeks (about 3 months) post-injection, subjects treated with UCMS cells displayed an approximate decrease in rotations by 60 to 90%. While untreated subjects demonstrated deteriorating performance and controlled subjects had no notable change in behavior following brain lesions, those treated with UCMS cells presented partial symptomatic relief (Weiss, Medicetty, et al.).
Transplantation of Patient-derived Induced Pluripotent Stem Cells without viral Transcription Factors
The transplantation of iPSCs serves as an alternative option for stem cell therapy in PD patients as well. Induced pluripotent stem cells (iPSCs) were first discovered by Kazutoshi Takahashi and Shinya Yamanaka in 2006. The discovery of human-iPSCs (hiPSCs) served as a significant advancement in developmental biology, as it demonstrated outstanding potential as stem cells that lack ethical implications and risk of immune rejection. Somatic cell reprogramming advanced through a multistep process: genetically altering the somatic cell nucleus, defining the cell culture conditions that allow isolation of embryonic stem cells from the blastocyst, and finally creating iPS cells functionally like ESCs (Schmidt and Plath). On the other hand, the transplantation of iPSCs in the human body has posed major difficulties, as clinical trials on mice have demonstrated frequent tumor formation on iPSC-derived chimeras that alter the reprogramming factors present in the stem cell (para 5).
To combat such limitations, an experiment was conducted using fibroblasts of patients with sporadic PD. 1*106 dermal fibroblasts of five patients with sporadic PD and two unaffected subjects were infected with active lentivirus and DOX-inducible lentiviruses to effectively transduce either four (OCT4, SOX2, c-MYC, KLF4) or three (OCT4, SOX2, KLF4) transcription factors. 3 to 5 weeks following the DOX-induced transgene expression, hiPSCs that demonstrated hESC-like morphology were selected. As a result, all fibroblasts extracted from PD and non-PD patients successfully became stable hiPSCs independent from DOX for more than 30 passages. The hiPSCs analyzed by quantitative RT-PCR displayed pluripotent reprogramming factors: Tra-1-60, SSEA4, OCT4, SOX2, and NANOG with comparable levels of expression in hESCs (Soldner, Hockemeyer, et al.)
Results
Effects of Transplanting Human Umbilical Cord Stem Cells
As a result, clinical trials have revealed that human umbilical cord stem cells, including HMCs, MSCs and cord mononuclear stem cells all contribute to symptomatic relief and increased survival rates in animal subjects. In the experiment conducted by Jackson Laboratory, mice treated with congenic bone marrow stem cells and mice treated with human mononuclear cord blood stem cells displayed a significant increase in survival rates compared to those untreated. While only 10% of the untreated mice survived, 20% of mice treated with congenic bone marrow and 33.3% of mice treated with human mononuclear cord blood stem cells survived. Furthermore, in research conducted by Weiss, Medicetty, etc., hemiparkinsonian rats treated with UCMS cells expressed notable symptomatic relief and behavioral changes. Rotations decreased by 60% to 90%, suggesting that UCMS cells are a viable source for treating Parkinson’s disease.
Morphology of post-transplantation hiPSCs in PD patients
In research conducted by Soldner, Hockemeyer, etc., results have revealed that hiPSCs also have significant therapeutic value in treating Parkinson’s Disease. While major side effects such as tumor formation in the brain prevent researchers from conducting clinical trials on the human body, projected results of the experiment have shown how all fibroblasts extracted from PD and non-PD patients transformed into stable hiPSCs post-DOX-induced transgene expression. Here, the newly formed hiPSCs remained independent from DOX for over 30 passages, and no viral reprogramming factors were present. Instead, they expressed extremely similar morphology to hESCs through the presence of pluripotent reprogramming factors such as Tra- 1-60, SSEA4, OCT4, SOX2 and NANOG.
Discussion
It can be understood from the methods that using stem cells to treat diseases is effective. Here, two types of stem cell therapies are introduced: treatments using umbilical cord stem cells and treatments using iPSCs. The results indicate that, although there is not a 100% chance of curing the disease, subjects treated with stem cell therapy show increased survival rates compared to those who did not receive any treatment. The analysis supports the theory that stem cells are more beneficial than conventional treatments. These results are in line with the claims made by Liu, Cheung. Contrary to the hypothesized belief, iPSCs aren’t the only viable method of stem cell production; the results contradict this, as iPSCs pose a higher risk of tumor formation. However, tackling that obstacle proved that human iPSCs were beneficial.
While numerous types of stem cells are available as potential sources of stem cell therapy in PD, umbilical cord blood stem cells and hiPSCs should be considered the two most viable options of stem cell therapy, considering how accessible, ethical, and effective they are. The results build on the existing evidence of how umbilical cord blood stem cells are not only effective in relieving symptoms but have been increasingly made accessible for patients. Despite the financial implications that may serve as a limitation for some patients, the unethical nature of the treatment suggests that they store immense potential. On the contrary, results have displayed that hiPSCs are also effective in treating the disease due to their pluripotent expression, such as embryonic stem cells. While hiPSCs also remain costly for some, they may be an ideal stem cell source for others, considering the lack of ethical implications.
However, the reliability of this data is impacted by the animal subjects, as one of the methods conducted experiments on mice. The anatomical and genetic differences between humans and mice affect the reliability of this experiment, especially when it comes to comparing the effectiveness of the procedure. Future studies should use this existing evidence as an incentive to conduct more human trials for further advancements in regenerative medicine.
Conclusion
Stem cells have proven their effectiveness in the trials conducted. Although they are a relatively new concept in the medical field, their promising performance in medical studies has made researchers investigate them more. Treatments have come a long way and have progressed over the years – treating and providing relief to patients diagnosed with Parkinson’s. Utilizing stem cell treatments would be a major advancement and provide effective, long-term relief for patients with PD. Their proliferative and regenerative properties would be highly beneficial in treating such degenerative diseases. Researchers at the Harvard Stem Cell Institute have generated neurons that produce dopamine by biologically converting adult skin cells into induced pluripotent stem cells and prompting them to become dopaminergic neurons.
Although there are ethical concerns and chances of rejection, stem cells derived from humans, such as the iPSCs, would have much lower risks and be more reliable and accurate in terms of results. There is still much to uncover about stem cells and their properties, but as of right now, iPSCs and mesenchymal stem cells are the two most promising and viable options. Stem cells have opened a huge window of possibility and have given hope of treating and perhaps even curing such diseases. With promising advancements and further trials, millions of lives could be saved. The future of regenerative medicine looks very bright.
References
Introduction:
UCLA Broad Stem Cell Research Center. “Induced Pluripotent Stem Cells (IPS) | UCLA Broad Stem Cell Center.” Ucla.edu, 2007, stemcell.ucla.edu/induced-pluripotent-stem-cells.
“Types of Stem Cells – a Closer Look at Stem Cells.” Closerlookatstemcells.org, 2019, www.closerlookatstemcells.org/learn-about-stem-cells/types-of-stem-cells/#tissue-specific.
“Embryonic Stem Cells – Stem Cells and the Future of Regenerative Medicine – NCBI Bookshelf.” National Center for Biotechnology Information, https://www.ncbi.nlm.nih.gov/books/NBK223690/#:~:text=Embryonic%20stem%20cells%20(ESCs)%20are,the%20three%20embryonic%20tissue%20layers
“Statistics | Parkinson’s Foundation.” Parkinson’s Foundation, https://www.parkinson.org/understanding-parkinsons/statistics
Parkinson’s Disease:
“Deep Brain Stimulation (DBS).” Cleveland Clinic, my.clevelandclinic.org/health/treatments/21088-deep-brain-stimulation
“Deep Brain Stimulation (DBS) for the Treatment of Parkinson’s Disease and Other Movement Disorders | National Institute of Neurological Disorders and Stroke.” Www.ninds.nih.gov, www.ninds.nih.gov/about-ninds/impact/ninds-contributions-approved-therapies/deep-brain-stimulation-dbs-treatment-parkinsons-disease-and-other-movement-disorders.
“Deep Brain Stimulation | Parkinson’s Disease.” Www.michaeljfox.org, www.michaeljfox.org/deep-brain-stimulation.
“Etiology of Parkinson’s Disease – an Overview | ScienceDirect Topics.” Sciencedirect.com, 2015, www.sciencedirect.com/topics/neuroscience/etiology-of-parkinsons-disease.
Gwinn, Marta. “Genetics, Coffee Consumption, and Parkinson’s Disease | CDC.” Www.cdc.gov, 28 Jan. 2019, www.cdc.gov/genomics/hugenet/casestudy/parkinson/parkcoffee_view.htm.
Kouli, Antonina, et al. “Parkinson’s Disease: Etiology, Neuropathology, and Pathogenesis.” Parkinson’s Disease: Pathogenesis and Clinical Aspects, 22 Dec. 2018, pp. 3–26, www.ncbi.nlm.nih.gov/books/NBK536722/, 10.15586/codonpublications.parkinsonsdisease.2018.ch1.
Mayo Clinic. “Parkinson’s Disease – Symptoms and Causes.” Mayo Clinic, 2020, www.mayoclinic.org/diseases-conditions/parkinsons-disease/symptoms-causes/syc-20376055.
Schapira, A. H.V. “Etiology of Parkinson’s Disease.” Neurology, vol. 66, no. Issue 10, Supplement 4, 22 May 2006, pp. S10–S23, n.neurology.org/content/66/10_suppl_4/S10, 10.1212/wnl.66.10_suppl_4.s10, www.n.neurology.org/content/66/10_suppl_4/S10.
Tysnes, Ole-Bjørn, and Anette Storstein. “Epidemiology of Parkinson’s Disease.” Journal of Neural Transmission, vol. 124, no. 8, 1 Feb. 2017, pp. 901–905, 10.1007/s00702-017-1686-y.
“Young-Onset Parkinson’s Disease | Johns Hopkins Medicine.” Johns Hopkins Medicine, Based in Baltimore, Maryland, 1 Nov. 2021, https://www.hopkinsmedicine.org/health/conditions-and-diseases/parkinsons-disease/youngonset-parkinsons-disease#:~:text=It’s%20not%20common%20to%20see,%2Donset%20Parkinson’s%2C%20or%20YOPD
Methods/Results:
Liu, Zhaohui, and Hoi-Hung Cheung. “Stem Cell-Based Therapies for Parkinson Disease – PMC.” PubMed Central (PMC), 21 Nov. 2020, https://www.ncbi.nlm.nih.gov/pmc/articles/PMC7663462/.
“L-DOPA – an Overview | ScienceDirect Topics.” ScienceDirect.Com | Science, Health and Medical Journals, Full Text Articles and Books., https://www.sciencedirect.com/topics/neuroscience/l-dopa
Schmidt, Ryan, and Kathrin Plath. “The Roles of the Reprogramming Factors Oct4, Sox2 and Klf4 in Resetting the Somatic Cell Epigenome during Induced Pluripotent Stem Cell Generation – PMC.” PubMed Central (PMC), 13 Oct. 2012, https://www.ncbi.nlm.nih.gov/pmc/articles/PMC3491406/.
Soldner, Frank, et al. Parkinson’s Disease Patient-Derived Induced Pluripotent Stem Cells Free of Viral Reprogramming Factors – ScienceDirect. 6 Mar. 2009, https://reader.elsevier.com/reader/sd/pii/S0092867409001512?token=0C3AB9E1E216ADEFE246F0814B0B179F18EB1095BB8FAEFBB70D4F21CF58CA750B5BDDC55F85C94A2BC2016D251D3FA6&originRegion=us-east-1&originCreation=20220812084642.
“Tyrosine Hydroxylase and Regulation of Dopamine Synthesis – PMC.” PubMed Central (PMC), https://www.ncbi.nlm.nih.gov/pmc/articles/PMC3065393/#:~:text=Tyrosine%20hydroxylase%20is%20the%20rate,in%20regulating%20the%20enzyme’s%20activity
Weiss, Mark, et al. “Human Umbilical Cord Matrix Stem Cells: Preliminary Characterization and Effect of Transplantation in a Rodent Model of Parkinson’s Disease | Stem Cells | Oxford Academic.” OUP Academic, Oxford University Press, 13 Oct. 2005, https://academic.oup.com/stmcls/article/24/3/781/6415888.
Ende, Norman, and Ruifeng Chen. “Parkinson’s disease mice and human umbilical cord blood.” Journal of medicine 33.1-4 (2002): 173-180
Conclusion:
“Trial Shows Promise of Stem Cells to Treat Parkinson’s | Cryo-Cell.” Cryo-Cell.com, 2021, www.cryo-cell.com/the-benefits-of-banking/cord-blood-cord-tissue-research/parkinson-treatment-promise-stem-cells.
Alanctot. “Induced Pluripotent Stem Cells: The Future of Tissue Generation – HOPES Huntington’s Disease Information.” HOPES Huntington’s Disease Information, 28 July 2011, hopes.stanford.edu/induced-pluripotent-stem-cells-the-future-of-tissue-generation/