Supervised by: Klara Hatinova BSc (Hons). Klara obtained a first in her Neuroscience undergraduate degree, which included a nomination for a year abroad at the University of California, Los Angeles. She is currently pursuing her MSc in Clinical and Therapeutic Neuroscience at the University of Oxford, working on a computational project which uses psychedelic medicine to treat depression.
Introduction
“Dementia is now the 7th leading cause of mortality globally and, as we know from previous World Alzheimer Reports, one of those with the highest cost to society.” (Zeisel et al, 2020). Alzheimer’s disease (AD), the most common form of dementia (Gandy, 2005), is defined as a progressive neurological disorder, characterized by a gradual decline in memory, language, cognitive, social and behavioral skills (Zeisel et al, 2020). The economic impact of AD is significant as it has been determined that almost $1.3 trillion per year has been spent on the care of AD patients, and that by 2030 the cost is predicted to increase to $2.8 trillion (Figure 1).
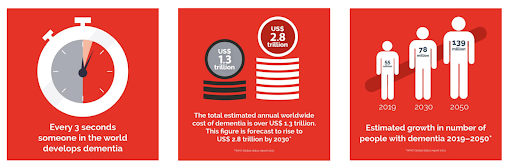
Figure 1: The prevalence of Alzheimer’s disease and its associated cost is increasing dramatically. Taken from Zeisel et al, 2020
The underlying neurobiology observed in AD suggests atrophy of cortical volume, which is a result of cell degeneration. In fact, the progressive loss of cognitive function and memory associated with AD is dependent on the loss of cerebral tissue functionality correlated with neural death (Gandy, 2005). The first neural population impacted are the cholinergic neurons in the midbrain, including the hippocampus and entorhinal cortex, which translates into behavioral deficits in memory being the first indicators of AD. There are two main hypotheses that explain what is causing the degeneration of cholinergic neurons, informed by neuroimaging and post-mortem studies.
The Amyloid Cascade and Tau Hypotheses
In the brain of an Alzheimer’s patient the predominant observation is the existence of senile plaques, which are deposits of a protein fragment called beta-amyloid (Aβ), and neurofibrillary tangles which are abnormal structures that have been caused due to changes in the tau protein which exists inside the body of nerve cells (Bloom, 2014). In accordance with the “amyloid cascade hypothesis”, (Hardy and Higgins,1992; Hardy and Selkoe, 2002) the accumulation of Aβ peptides exhibits the pathogenesis of AD. Symptoms that are displayed by AD patients are caused by neural cell degeneration and neural death whereby impairment begins in the regions of the brain which are responsible for memory and language, that will then successively progress and spread to the entire brain. The current method of treating AD patients is by suppressing and removing formations of amyloid plaques or neurofibrillary tangles in the brain.
Tau is a neuronal microtubule-associated protein which binds to the axonal microtubules in order to stabilize them. If the tau proteins become phosphorylated, it causes separation with the microtubules consequently forming paired helical filaments in the neural cytoplasm (Iqbal et al, 1998). Researches have been completed to find out whether Aβ has a role in forming neurofibrillary tangles. For example, (Gotz et al, 2001) examined the impact of injecting Aβ into the brain of a tau transgenic mouse. Results showed that there was a 5-fold increase in the formation of neurofibrillary tangles that was prompted by an increase in tau phosphorylation.
What are stem cells, where do they come from and what types are there?
Stem cells are cells with unlimited multiplication capacity and the ability to form multiple cell types. This property has been used in multiple research fields to investigate the molecular and genetic causes of disease in vitro, as well as potential therapeutic applications. In neuroscience, induced pluripotent stem cells (iPSCs) can be transformed into a neural model that reflects the patient’s genetic background and investigate amyloid beta and tau aggregation. Moreover, these cells have been used to screen for therapeutic safety and efficacy. Multiple stem cell types have been investigated for their therapeutic properties when implanted into the brain. These include embryonic stem cells (ESC, derived from the blastocyst), mesenchymal stem cells (MSC, derived from the blood of the umbilical cord) and neural stem cells (NSC), which can be induced from iPSCs. In this review, we will focus on the applications of different types of stem cells for examining the genetic and molecular biology of Alzheimer’s disease as well as using stem cell implantation as a new direction in AD treatment.
The genetic mechanics
There are three single-gene mutations associated with early-onset AD including amyloid precursor protein (APP) on chromosome 1, presenilin 1 (PSEN1) on chromosome 14 and presenilin 2 (PSEN2) on chromosome 1. Even if the cause of Alzheimer’s is not known, the disease manifests itself after aggregation of toxic proteins in the brain. The present hallmark pathological features are senile plaques (deposits of a distinct protein frag) and neurofibrillary tangles (abnormal structures formed by changes in the tau protein inside nerve cell bodies).
The major component of amyloid plaques in the AD brain is the amyloid-beta or Aβ and the most common form of it is Aβ40 (40 amino acids), but an accumulation of Aβ-42 harms the neurons. The apolipoprotein E gene (APOE) is divided into three categories: E2, E3 and E4. One of these types, E4, is often associated with AD, though it is not known if it can be linked directly to the cause. On the other hand, E2 and E3 are connected to providing protection against Alzheimer’s disease and since everyone possesses the APOE gene, it opens the possibility of gene editing in order to find a cure.
iPSCs have also been used to study the underlying molecular neurobiology of AD.
Studies have examined the way in which donor-derived iPSC has been used in order to replicate in vivo molecular signatures of AD. Due to the lack of understanding around the concept of clinical onset and pathogenesis of sporadic AD caused by the scarcity of access to brain-derived neuronal cells (which have been troubled with neurodegenerative diseases), the development of in-vitro models reflecting brain neurons is essential. The result of this research has evidenced that dermal fibroblasts derived from a patient with sporadic AD patients can be induced into a pluripotent state (Hossini et. al, 2015). Even though researchers have identified the clear role that Aβ plays in the pathogenesis of AD, the mechanism involved is not yet determined. By generating iPSC-s from familial and sporadic AD patients and differentiating them into neural cells, we are able to study the development of the pathological processes in a human genetic background. Thus, patient-specific iPSCs can be utilized in further analysis of AD pathogenesis and evaluation of drugs (Takayuki kondo et. al, 2013). Nonetheless, there is more work to be done to discover more applications and increase the translatability of these iPSC models.
The pharmaceutical response to stem cell treatment
Currently, there have been several medications approved for Alzheimer’s disease but less than 20% of the known cases have seen some improvement. Stem cells have been used to check the safety and efficacy of early-stage drugs. For example, Yahata et al (2011) found that secretase inhibitors were able to inhibit (Aβ) accumulation in iPSCs. However, the ability of the screened compounds to do so depended on the development stage of the iPSC, questioning the effect of these compounds when applied to humans.
Another study, based on a drug screening method, conducted by researchers from the University of California-San Diego takes a different approach in order to find the cause of failure for previously attempted treatments. This method, led by professor Shankar Subramaniam and his colleagues, attempted to observe the changes in a patient’s neurons after a treatment. This method consisted of taking human induced pluripotent stem cells derived from patients with familial Alzheimer’s disease and transforming them into neurons, which are then treated with the respective drugs. The conclusion researchers came to was that the drugs they were testing only mended some endotypes so now they have a clear idea of the endotypes that need to be targeted(Caldwell et al, 2022).
Given the complex nature of neurodegenerative diseases, there aren’t any competent pharmaceuticals available at the moment but numerous studies have been conducted, showing that there could be similar potential factors in different patients. The theory that towers over the rest of the potential ones is the “Amyloid Cascade Theory”, but the accumulation of amyloid is not correlated with behavioral deficits (Hyman, 2011). When talking about conceiving supposed medication, it has been considered to culture neurons from various patients in order to help searching for better treatment, since a personalized cure might have a higher effect.
Using stem cells in AD treatment
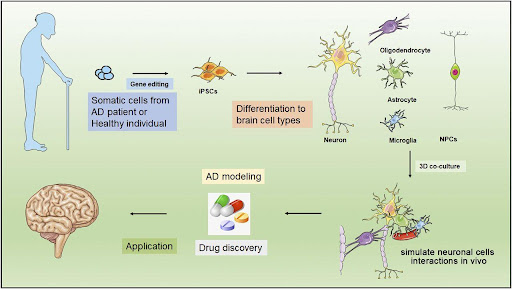
Figure 2: Potential uses of stem cells in Alzheimer’s disease therapy and modeling. Taken from (Si & Wang, 2021)
A commonly known medication that is used to treat Alzheimer’s is Aducanumab. This particular form of medication is the only disease-modifying immunotherapeutic medication that is approved to treat Alzheimer’s (Selkoe, 2019). Aducanumab uniquely behaves as a human antibody by targeting the protein beta-amyloid to help reduce amyloid plaques. This medication is predominantly prescribed to AD patients who show symptoms of mild cognitive impairment or mild Alzheimer’s. However, as stated previously, a large proportion of patients do not respond to these treatments, indicating a need to find new strategies to tackle the cognitive and behavioral impairment of AD patients. For this purpose, stem cells present a unique, novel solution which is attracting attention in the research community.
Neural stem cells
Brain-derived NSCs are multipotent cells that are responsible for generating all types of neural cells during development. They can give rise to neural progenitor cells that can develop and differentiate into neurons and glial cells. For example, Asymmetric NSC division produces a variety of cell types that replace injured neurons, and neurotrophic factors generated by differentiated cells and stem cells are linked to fast differentiation and play a key role in neuroprotection to restore synaptic density. The multifaceted therapeutic actions of NSCs could therefore greatly benefit future treatment of Alzheimer’s Disease (Boese et al, 2020).
While NSC’s exist in the adult brain as well, they are restricted to the subventricular zone’s distinct neurogenic niches and the granular layer of the dentate gyrus in the hippocampus. For many years, the brain was assumed to be a closed, fixed system, into which no new neurons are added during life (Duncan & Valenzuela, 2017). NSC therapy, however, offers great promise for CNS disorders, such as AD. In addition to the replacement of dead or dying brain cells, NSCs release therapeutic gene products and generate a favorable milieu for the survival of host cells and appropriate CNS function in addition to replacing dead or dying brain cells (Boese et al, 2020)
Continuously, NSCs can be collected from brain tissue or genetically reprogrammed from somatic cells. By transplanting NSCs, they target both neuron networks and pathogenic proteins, which is a potential treatment method that improves the microenvironment to increase cell survival and thereby improve cognitive performance (Hermann & Storch, 2013; Shahbazi et al, 2018). NSCs can also be differentiated from embryonic stem cells (ESCs) and iPSCs (Martínez-Morales et al, 2013). As with drug therapy, NSC transplantation appears to enhance cognitive behavior in animal models of Alzheimer’s disease, Parkinson’s disease, Huntington’s disease, amyotrophic lateral sclerosis and other neurodegenerative disorders (Martínez-Morales et al, 2013). Furthermore, transplantation can also decrease neuroinflammation, a hallmark of Alzheimer’s disease, which usually alters extracellular pH and causes structural changes in AD, making it more difficult to remove from the CNS.
NSCs can be transplanted into the brain and improve the spatial navigation skills as well as memory of mice via BDNF release. As an example, A-beta is a large membrane protein that normally plays an essential role in neural growth and repair. However, later in life, a corrupted form can destroy nerve cells, leading to the loss of thought and memory in Alzheimer’s disease. NSCs, however, can overexpress A-beta degrading enzymes that will be able to increase the clearance of pathological A-beta fragments (Hayashi et al, 2020).
Novel medications in development are now focusing on targeting these processes in order to slow or reverse the disease progression. NSC therapy is a potential and adaptable innovative therapeutic option for tackling the underlying cause of AD since it can restore damaged cells, reduce A aggregation, improve AD pathology, and restore neuronal cell populations (Wu et al, 2016). Unfortunately, efficacy in comparison to placebo groups has been inconsistent, and there have been various ethical problems and differences about how they should be treated properly (Kwak et al, 2018). Stem cell treatment, on the other hand, is unquestionably one of the most promising therapeutic techniques currently under investigation.
Blurton-Jones et al (2009) evaluated the effect of neural stem cell transplantation on AD-related neuropathology and cognitive dysfunction. They employed aged triple transgenic mice (3xTg-AD) that produce pathogenic versions of amyloid precursor protein, presenilin, and tau. This finding demonstrates that neural stem cells can ameliorate complex behavioral deficits associated with widespread Alzheimer’s disease pathology via BDNF. The loss-of-function studies show that depletion of NSC-derived BDNF fails to improve cognition or restore hippocampal synaptic density. And due to a significant increase in hippocampal synaptic density, which is mediated by a brain-derived neurotrophic factor (BDNF), it increases cognition. As a result, their cognitive function is improved without altering Aß or tau pathology and restores hippocampal synaptic density. The recombinant BDNF mimics the beneficial effects of NSC transplantation. Thereby, neural stem cells enhance the brain’s self-renewing capacity to counteract cell loss as a result of AD pathology.
Embryonic stem cells
Embryonic stem cells are pluripotent stem cells (a cell that can develop into many different types of cells or tissues in the body). These are derived from the inner mass of the blastocyst; an early-stage embryo. This stage is 4-5 days post fertilization – consists of 50-150 cells approximately. Transplants show hope to restore cognitive function in animal models of brain injury, however, clinical studies have been limited due to their nature, such as transplantation of undifferentiated embryonic stem cells- this presents an inherent risk of uncontrolled cell growth and tumor formation (Duncan and Valenzuela, 2017). However, if you differentiate ESC to NSC through Vitro pre-differentiation, it circumvents some of these risks as this generates cholinergic neurons which induce improvements in spatial memory performance post- transplantation with the AD rodent model (Duncan and Valenzuela, 2017).
The study into the cholinergic neurons for cell restorative therapy in the rodent AD model using ESC. The behavioral assessment of the rodents using Morris water maze and spatial probe test revealed a significant behavioral improvement in memory deficits following transplantation. It was concluded that 40% of rodents used had received the cholinergic cell phenotype and had follow up after surgery and concluded no tumor formation- an inherent risk of ESC transplants. This indicates that these may be safe for human transplantation, with many more rodent experiments and then clinical trials (Moghadam et al, 2009) . This study has important implications as it suggests that the transplantation of mouse ESC following commitment to a cholinergic cell phenotype can promote behavioral recovery in a rodent model of AD (Moghadam et al, 2009).
Recently, another study reported the stable generation of cholinergic neuronal populations from human ESC’s. Following on after transplantation, these were able to functionally integrate into hippocampal neuronal circuitry. In 2013, a study reported the conversion of ESC’s into medial ganglionic eminence, a transient stem cell type present in the developing brain (Duncan and Valenzuela, 2017). After the transplant, the cells were capable of maturing into both GABAergic and cholinergic neuronal subtypes and synaptically integrating with host neuronal circuits (Duncan and Valenzuela, 2017). This leads to improvements in the impaired spatial memory and the learning. Despite their success, there are implicit ethical and immunogenic limitations to using allogeneic donor cells that significantly hamper the clinical translation of ESC-based therapies (Duncan and Valenzuela, 2017).
A human trial was also conducted to evaluate the effect of Alzheimer’s patient serum on neural gene expression in mESC-derived neural cells; plated EBs were treated in two groups. In the first group, plated EBs cultured in a medium containing 5% serum from a healthy individual as a control group or 5% serum from Alzheimer’s patients (Dehghani et al, 2013). Then 7 days post-plantation neural gene expression was assessed and compared in both groups. The reduction in neurite outgrowth was observed in neural cells in the group which were treated with serum from Alzheimer’s patients while neurite growth was natural in appearance in the control group. The two groups were then compared and Nestin expression did not significantly differ among the groups (Dehghani et al, 2013).
Mesenchymal stem cells
A wide variety of studies and research has been done on the impact of using Mesenchymal stem cells as a method to treat Alzheimer’s. Mesenchymal Stem Cells (MSCs), classified as multipotent, are harvested from a variety of tissues, but most commonly from umbilical cord blood (UCB-MSCs), as well as Wharton’s Jelly, bone marrow and adipose tissue. MSCs are among the most investigated stem cell types because of their accessibility, relative simplicity in handling, and ability to create a diverse spectrum of cell types (Duncan & Valenzuela, 2017). Thus, their ability to differentiate into a multitude of mesenchymal lineages (adipocytes, osteocytes and chondrocytes), but most relevantly neural cells, allows a chance of treating the neurodegenerative disorder, Alzheimer’s. (U.S. National Library of Medicine, 2022)
While the efficacy of MSC’s abilities to differentiate into neuronal cells in vivo and in vitro holds promise, it’s still being questioned, and a lot remains to be known. These Stem cells possess several properties key to treating Alzheimer’s disease (AD), beginning with their ability to activate microglial cells (cells that remove damaged neurons), and thereby clear deposits of Amyloid Beta build-up, which is considered the root cause of AD (Figure 3 (Hamisha et al, 2015)). Since the cells can originate from bone marrow, they can even activate additional microglial cells from bone marrow, through the expression of the gene CCL5 (Duncan & Valenzuela, 2017).
Additionally, it’s important to indicate the fact that MSCs also have the potential to increase endogenous neurogenesis—process of new neurons forming in the brain— modulate neuroinflammation, reduce levels of β-secretase, and tau hyperphosphorylation, stimulate anti-inflammatory cytokines, and offer neuroprotection (Figure 3 (Hamisha et al, 2015; Hernández & García, 2021). This is vital, as neurogenesis can counteract the deterioration of neurons, as happens in Alzheimer’s patients, and act as a “compensating mechanism”, for the effects of the Amyloid Beta buildup. The MSC is engrafted into the Central Nervous System, most observably the hippocampus, but also the choroid plexus and other sites, protecting the CNS from AB plaque. In fact, a study involving Aβ25–35 oligomers injection followed by MSC Stem Cell Administration, in the lateral ventricle of mice, has shown an usual increase in the number of new neurons formed, as well as an usual increase in the stimulation of hippocampal neurogenesis in the animals. It also demonstrated how the MSC cells prevented cognitive defects otherwise caused by the Amyloid plaque, because they have activating microglial cells, and reducing the plaque build-up (Hamisha et al, 2015). Thus based on this research, MSCs have a high potential to inhibit the progression of Alzheimer’s.
Mesenchymal stem cells
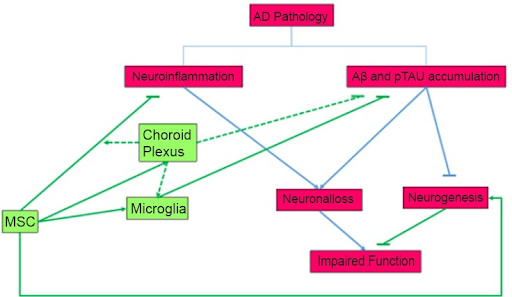
Figure 3: This diagram shows the impact of MSC’s on triggering neurogenesis, and activating microglia, and their relationship to decreasing neuroinflammation, and Ab and Tau accumulation and understanding AD Pathology. Taken from (Hamisha et al, 2015).
There have been many studies and clinical trials on this topic, but only a few human ones have been completed. A recent human phase 1 clinical trial involved subjects with mild-to-moderate Alzheimer’s diseases all receiving a single stereotactic brain infusion of human umbilical cord mesenchymal stem cells. Three patients were given a single low dose infusion, while six were given a high dosage infusion. During the two-year follow-up, the injection into the hippocampus was found to be safe, feasible and well-tolerated, with no significant side effects, but it didn’t produce the same level of therapeutic impact as shown in animal trials. The single USB-MSC injection had very minimal efficiency in improving the physiology of AD. The differences between animal and human trials could account for different AD microenvironments, and the different types of transplantation (allogenic vs xenogenic) and scanning methods for humans rather than animals (Hernández & García, 2021)
Another notable phase l trial involves intracerebroventricular injection of umbilical cord human mesenchymal stem cells into Alzheimer’s patients. 9 patients participated, with 3 receiving a low dose, 6 receiving a high dose, and the injection repeated three times. After the follow-ups, the most common side effect was a fever, but overall there were no complications involving tumors or hemorrhaging, and the study was found to be safe and feasible. There were reduced levels of Tau, and Ab after day 1, but then after 4 weeks, there was again an increase, suggesting that the HUB-MSCS don’t have a prolonged efficacy. However these are only phase l trials, so they only were designed to test for the safety of the stem cells in AD subjects (Hernández & García, 2021). Mostly, there have been preclinical studies in rodents, and all human trials progressing, past phase 1, are still in progress.
Compared to the other types of Stem Cells, Mesenchymal ones pose a lower risk of inducing cancer cells, however, we need to consider the limitations on them. One crucial factor is MSC cellular heterogeneity, which makes drawing consistent conclusions about MSC therapeutic potential challenging because the results obtained are usually diverse and may depend on the different MSC origins as well as harvesting processes. Another challenge is long term, the MSC’s differentiation potential, still poses the risk of de-differentiation or transforming into a tumorous cell long term. So while there’s a lot of research already done on the use of Mesenchymal Stem Cells for Alzheimer’s, there’s still a lot to discover, and a long way to go for it to be effective on humans (Musiał-Wysocka et al, 2019).
Induced pluripotent stem cells
Induced Pluripotent Stem Cells, or iPSCs, are a type of pluripotent cell that can be derived from the skin or blood cells. IPSCs enable the development of any type of human cells needed for therapeutic needs. Stem cells like these can be useful when treating neurodegenerative disorders such as Alzheimer’s Disease (AD). A significant amount of research has been done already regarding iPSCs and the ways they help people with AD. For example, using iPSC technology to create AD models. Although a lot of progress has already been made, there are always challenges that lie on the road ahead to perfect AD modeling, iPSC research, and to finding the long term solution to AD (Doss & Sachinidis, 2019).
Mice are the best animals to use for AD modeling as they have the closest evolutionary relationship to human beings, and since genetic mutation can alter the way that disease progresses, it is important to have mouse models reflect that. (Jankowsky and Zheng, 2017; Penney et al, 2020). Some limitations for mouse models are the fact that when a mouse mutation demonstrates human diseases and mutations it rarely results in neurodegenerative phenotypes. This is because the greatest commonality between patients with Alzheimer’s is their age, as people usually develop their symptoms in the later half of their life, around 60 years or so. This is an issue when using mouse models, as mice have a significantly shorter life span than humans do (Penney et al, 2020). AD models using iPSC generated from FAD (familial AD) and SAD (sporadic AD) genes have already been established and have helped make discoveries. In the same way, it is important to use iPSCs generated from healthy individuals to compare the healthy and diseased cells. This can help identify cellular phenotypes associated with a disease. (Penney et al, 2020; Yang et al, 2016). From applying iPSCs to AD modeling it has been found that forebrain cholinergic neutron (FBCN) loss can be connected to some of the memory issues in AD. This means that the generation of FBCNs from iPSCs, that come from AD specific patients is essential for modeling and possibly for the future of t developing AD therapies. (Yang et al, 2016).
An example of the progress of iPSC research throughout the years, is research conducted in 2006 by Shinya Yamanaka and his associates. They found that fibroblast cells in mice can be reprogrammed into iPSCs. This can happen by using four different transcription factors known as Oct4, Sox2, Kruppel-like factor 4, and cMyc (Yagi et al, 2012). In 2007, these discoveries made it so that the technology could be applied within human somatic cells. It was also made so the combination of the 4 transcription factors could induce iPSC from the human somatic cells as well (Yagi et al, 2012; Yang et al, 2016). The induction of iPSCs has been made to include different types of donor cells. A few examples are liver cells, stomach cells, fibroblasts, blood cells, and many more (Yang et al, 2016). The delivering methods for inducing iPSC have also been optimized. The issue before optimization was that the random integration of transgenes into the genome causes the chance of tumor formation and death in the mice that were derived from iPSCs. To optimize the situation, Pingping Hou and associates reported that molecules like VC6T plus FSK can replace “master genes” Sox2 and Oct4 for inducing cell reprogramming (Li et al, 2011; Yang et al, 2016).
Although so much research has been done, there are still many unknowns and there is always more research that can be done about iPSCs and AD modeling. For instance, something that is still misunderstood is the molecular principles of using iPSCs for disease modeling, especially in human cells. The reason for this is using cells derived from different individuals, or otherwise known as donor-to-donor variability. With more time and research, iPSC AD models will be able to improve greatly and will be able to help many people with AD.
Conclusion
Treatment using stem cells is a new and promising field that researchers are just starting to focus on. Its utility in the coming years has been demonstrated in numerous studies, including for the investigation and therapy of Alzheimer’s. The self-reproducing and differentiation potential of stem cells makes them a malleable and accessible model. iPSCs and other human derived stem cells can help in expanding the study of genetics and the identification of Alzheimer’s disease, thanks to their human background. While the use of some stem cell types is linked to ethical issues, and potential rejection from host patients, research progress has been working hard to overcome those limitations.
Even with so many studies, there is still a lot to discover, and there is always more research on SC and AD modeling that can be done. We found an expanding field of research using iPSCs to study molecular features of AD. In the therapeutic aspect, neural, mesenchymal and iPSCs are the most promising cell types that are currently being pursued in clinical trials. Hence, stem cells present a unique platform to study and alleviate the societal and healthcare burden of AD and improve the lives of billions of people affected by this neurodegenerative condition.
References
Blurton-Jones, M., Kitazawa, M., Martinez-Coria, H., Castello, N. A., Müller, F. J., Loring, J. F., Yamasaki, T. R., Poon, W. W., Green, K. N., & LaFerla, F. M. (2009). Neural stem cells improve cognition via BDNF in a transgenic model of Alzheimer disease. Proc Natl Acad Sci U S A, 106(32), 13594-13599. https://doi.org/10.1073/pnas.0901402106
Boese, A. C., Hamblin, M. H., & Lee, J.-P. (2020). Neural stem cell therapy for neurovascular injury in Alzheimer’s disease. Experimental Neurology, 324, 113112. https://doi.org/https://doi.org/10.1016/j.expneurol.2019.113112
Caldwell, A., Liu, Q., Zhang, C., Schroth, G., Galasko, D., Rynearson, K., Tanzi, R., Yuan, S., Wagner, S., & Subramaniam, S. (2022). Endotype reversal as a novel strategy for screening drugs targeting familial Alzheimer’s disease. Alzheimer’s & dementia. https://doi.org/10.1002/alz.12553
Dehghani, L., Hashemi-Beni, B., Poorazizi, E., Khorvash, F., Shaygannejad, V., Sedghi, M., Vesal, S., & Meamar, R. (2013). Evaluation of neural gene expression in serum treated embryonic stem cells in Alzheimer’s patients. J Res Med Sci, 18(Suppl 1), S20-23.
Doss, M. X., & Sachinidis, A. (2019). Current challenges of iPSC-based disease modeling and therapeutic implications. Cells, 8(5), 403.
Duncan, T., & Valenzuela, M. (2017). Alzheimer’s disease, dementia, and stem cell therapy. Stem Cell Research & Therapy, 8(1), 111. https://doi.org/10.1186/s13287-017-0567-5
Gandy, S. (2005). The role of cerebral amyloid β accumulation in common forms of Alzheimer disease. The Journal of clinical investigation, 115(5), 1121-1129.
Hamisha, K. N., Tfilin, M., Yanai, J., & Turgeman, G. (2015). Mesenchymal stem cells can prevent alterations in behavior and neurogenesis induced by Aß25–35 administration. Journal of Molecular Neuroscience, 55(4), 1006-1013.
Hayashi, Y., Lin, H.-T., Lee, C.-C., & Tsai, K.-J. (2020). Effects of neural stem cell transplantation in Alzheimer’s disease models. Journal of Biomedical Science, 27(1), 29. https://doi.org/10.1186/s12929-020-0622-x
Hermann, A., & Storch, A. (2013). Induced neural stem cells (iNSCs) in neurodegenerative diseases. J Neural Transm (Vienna), 120 Suppl 1, S19-25. https://doi.org/10.1007/s00702-013-1042-9
Hernández, A. E., & García, E. (2021). Mesenchymal Stem Cell Therapy for Alzheimer’s Disease. Stem Cells International, 2021, 7834421. https://doi.org/10.1155/2021/7834421
Hyman, B. T. (2011). Amyloid-Dependent and Amyloid-Independent Stages of Alzheimer Disease. Archives of Neurology, 68(8), 1062-1064. https://doi.org/10.1001/archneurol.2011.70
Kwak, K.-A., Lee, S.-P., Yang, J.-Y., & Park, Y.-S. (2018). Current Perspectives regarding Stem Cell-Based Therapy for Alzheimer’s Disease. Stem Cells International, 2018, 6392986. https://doi.org/10.1155/2018/6392986
Li, Y., Zhang, Q., Yin, X., Yang, W., Du, Y., Hou, P., Ge, J., Liu, C., Zhang, W., Zhang, X., Wu, Y., Li, H., Liu, K., Wu, C., Song, Z., Zhao, Y., Shi, Y., & Deng, H. (2011). Generation of iPSCs from mouse fibroblasts with a single gene, Oct4, and small molecules. Cell Research, 21(1), 196-204. https://doi.org/10.1038/cr.2010.142
Martínez-Morales, P. L., Revilla, A., Ocaña, I., González, C., Sainz, P., McGuire, D., & Liste, I. (2013). Progress in stem cell therapy for major human neurological disorders. Stem Cell Rev Rep, 9(5), 685-699. https://doi.org/10.1007/s12015-013-9443-6
Moghadam, F. H., Alaie, H., Karbalaie, K., Tanhaei, S., Esfahani, M. H. N., & Baharvand, H. (2009). Transplantation of primed or unprimed mouse embryonic stem cell-derived neural precursor cells improves cognitive function in Alzheimerian rats. Differentiation, 78(2-3), 59-68.
Musiał-Wysocka, A., Kot, M., & Majka, M. (2019). The pros and cons of mesenchymal stem cell-based therapies. Cell transplantation, 28(7), 801-812.
Selkoe, D. J. (2019). Alzheimer disease and aducanumab: adjusting our approach. Nature Reviews Neurology, 15(7), 365-366. https://doi.org/10.1038/s41582-019-0205-1
Shahbazi, E., Mirakhori, F., Ezzatizadeh, V., & Baharvand, H. (2018). Reprogramming of somatic cells to induced neural stem cells. Methods, 133, 21-28. https://doi.org/10.1016/j.ymeth.2017.09.007
Si, Z., & Wang, X. (2021). Stem Cell Therapies in Alzheimer’s Disease: Applications for Disease Modeling. Journal of Pharmacology and Experimental Therapeutics, 377(2), 207-217. https://doi.org/10.1124/jpet.120.000324
Wu, C.-C., Lien, C.-C., Hou, W.-H., Chiang, P.-M., & Tsai, K.-J. (2016). Gain of BDNF Function in Engrafted Neural Stem Cells Promotes the Therapeutic Potential for Alzheimer’s Disease. Scientific reports, 6(1), 27358. https://doi.org/10.1038/srep27358
Yagi, T., Kosakai, A., Ito, D., Okada, Y., Akamatsu, W., Nihei, Y., Nabetani, A., Ishikawa, F., Arai, Y., & Hirose, N. (2012). Establishment of induced pluripotent stem cells from centenarians for neurodegenerative disease research. PLOS ONE, 7(7), e41572.
Yahata, N., Asai, M., Kitaoka, S., Takahashi, K., Asaka, I., Hioki, H., Kaneko, T., Maruyama, K., Saido, T. C., Nakahata, T., Asada, T., Yamanaka, S., Iwata, N., & Inoue, H. (2011). Anti-Aβ Drug Screening Platform Using Human iPS Cell-Derived Neurons for the Treatment of Alzheimer’s Disease. PLOS ONE, 6(9), e25788. https://doi.org/10.1371/journal.pone.0025788
Yang, J., Li, S., He, X.-B., Cheng, C., & Le, W. (2016). Induced pluripotent stem cells in Alzheimer’s disease: applications for disease modeling and cell-replacement therapy. Molecular Neurodegeneration, 11(1), 39. https://doi.org/10.1186/s13024-016-0106-3
Zeisel, J., Bennett, K., & Fleming, R. (2020). World Alzheimer report 2020: design, dignity, dementia: dementia-related design and the built environment.