Supervised by: Faissal Sharif BSc, MSc, DPhil cand. (Oxon). Faissal obtained a BSc in Biomedical Sciences at Maastricht University with a semester abroad at the Chinese University of Hong Kong. As a visiting scholar at the University of California, Irvine, he investigated biomarkers for Alzheimer’s Disease. He completed his MSc in Translational Neuroscience at Imperial College London. Beyond his work on psychiatric disorders and mental health, Faissal has developed an interest in the connection between medicine and technology through working with Medtech and Digital Therapeutic startups and organizations such as the European Space Agency. Currently, he is completing his DPhil at Oxford University in Clinical Neuroscience, focusing on novel neurotechnology to fight mental disorders.
ABSTRACT
Memory is an essential part of human cognition, since it allows individuals to frame their understanding of and behaviour within the present by recalling and drawing upon past events. This allows us to continue gaining information on skills and knowledge and is one of the most crucial traits for humans. Memory is currently defined in psychology as the faculty of encoding, consolidation, and retrieving information (Squire, 2009). With the presence of theorised neurons called “engrams”, memory is stored and received. Neuroplasticity is what allows a memory to consolidate in the human brain. Psychologists have further shown that memory can be classified into 3 categories, which are sensory, short-term and long-term. Long-term memory can be further divided into implicit memory (the subconscious recall of information) and explicit memory (the conscious recall of information). These memories are distributed across neuronal circuits that link to one another throughout the brain. To ensure efficient synaptic transmission, synaptic pruning occurs. This is when often-utilised synapses are strengthened and protected while reducing or removing less-frequently used synapses.
This subject poses a lot of unknowns, similar to a big memory gap, which this research paper will be discussing . A lot of knowledge gaps in amnesia remain in its symptomatology. Beyond just memory loss, the long-term effects and implications of amnesia on overall cognitive functioning and mental health aren’t clear as individuals with amnesia may experience various cognitive deficits beyond memory impairments. There are various types of amnesia that may involve different underlying processes. Retrograde amnesia, the most commonly portrayed version, refers to the loss of previously stored information. In contrast to this, anterograde amnesia refers only to the memory inaptitude to hold onto information after the diagnosis. Another point of interest is why amnesia doesn’t appear to affect skills acquired by the patient, also known as implicit memory. A lot of what has been discovered regarding memory is credited to the study of “Patient HM”, who underwent surgery to remove parts of his brain that were relevant to memory formation. Whilst amnesia refers to the loss of memory, some individuals are gifted with extraordinary memory capacity. For example, individuals with savant syndrome or London cab drivers.
Amnesia is one of the main causes of memory loss over time, and 1.8% of Americans gain dissociative amnesia on a yearly basis (Hull, 2023). As it is responsible for so many cases, it is imperative that we learn more about the condition to understand how the disease progresses and which mechanisms are involved. The lack of understanding and treatment options warrants further scientific inquiry.
In this research paper, we dive deeper into the science of memory formation and the types and mechanisms of amnesia, in hopes to gain insights into the secrets of memory.
INTROduction
The study of memory loss, particularly amnesia, was not always considered a scientific field or a medical condition. It had long been observed from many perspectives: spiritual, physical, and emotional. It was only in 1763 that Sauvages classified amnesia as a medical disorder, thus establishing its recognition as a distinct part of science (Langer, 2019). Today, amnesia is understood to be the loss of memories, containing facts, information and experiences (Mayo Clinic, 2022). Our memory can be classified into two categories: short-term memory and long-term memory, and the latter extends to explicit and implicit memories. In the early 19th century, clinical descriptions of amnesia appeared internationally in medical dictionaries and scientific encyclopaedias (Langer, 2019). When we form memories, our brain goes through two important stages: encoding and consolidation. During these stages, information flows from the sensory and association areas in the cortex to the hippocampus. As we receive new memories, the brain reshapes itself through a process called synaptic pruning, which involves neurotransmitters and neurons working together in an electrochemical system. The medial temporal lobe (MTL) is a vital part of our brain that includes the hippocampus and amygdala. It plays a crucial role in forming explicit memories. One well-known case study involved ‘Patient H.M.’, whose hippocampus and amygdala were removed. As a result, he was unable to form new memories but retained his implicit memory despite suffering from amnesia (Squire, 2009) . Currently, there are no treatments available for most types of amnesia. However, studies conducted on mice have shown that light can help restore lost memories, providing new insights into amnesia. The motive of this paper is to review several key points about amnesia and enhance the reader’s knowledge of the condition. Amnesia affects some kinds of memory more than others, specifically explicit memory, which includes information about previous experiences and factual knowledge, like the names of people. As noted before, implicit memory is an unconscious type of memory that pertains to acquired skills and habits, such as bike riding. Research has shown that implicit memory is less severely affected by amnesia than explicit memory. The reasons behind the difference in the damage that exists between these two memory types are important to explore. Additionally, identifying the cause of amnesia is crucial to help prevent it. Amnesia is caused by an amalgamation of several factors, but which factors put a higher risk than others? Even with advancements in neuroscience, no cure for amnesia has been found, and the complexity of the hippocampus and amygdala’s fragile nature makes it unlikely. Whilst therapy is an option, what other medicines work?
THE SCIENCE OF MEMORY FORMATION
Medial Temporal Lobe and Neurotransmitters
The medial temporal lobe (MTL) is a brain region that is known to mediate functions of episodic memory and learning (Das et al., 2016). It helps to coordinate learning and retrieval from the neocortex, which is responsible for attention, thought, and perception (Foerde, 2010). The MTL includes the amygdala, hippocampus, and parahippocampal regions, and is crucial for episodic and spatial memory (Sanchez, 2017). The hippocampus is believed to be a map for spatial localization. The memory function of the medial temporal lobe consists of processes including encoding, consolidation, and retrieval. Encoding is the process of how information perceived by our senses is converted into a memory trace, which is theorised as the way that memory is physically stored in the brain. Consolidation is how memory traces are stabilised. Retrieval refers to the process of how memory traces are reactivated to access previously encoded information stored in the brain (Sanchez, 2017). If there was damage to the hippocampal region or other MTL areas, such as the cortices, new learning would be impaired (anterograde amnesia), and memory for information before the damage occurred would also be impaired (retrograde amnesia). Scientists wanted to know if damage to the MTL can cause ungraded memory loss more so than graded retrograde amnesia. Some researchers concluded that retrograde amnesia is temporally ungraded and that recent and remote memories are similarly impaired. However, other researchers concluded that retrograde amnesia is temporally limited and related to the extent and locus of the damage (Bayley et al., 2006). Although the medial temporal lobe is a major part of understanding memory, neurotransmitters are another big part of how memory is maintained.
Neurotransmitters are chemical messengers that your body cannot function without. They carry messages from one nerve cell (neuron) to the next target cell. There are three types of neurotransmitters: excitatory, inhibitory, and modulatory. Excitatory neurotransmitters “excite” the neuron and cause it to pass off the message to the next cell. Inhibitory neurotransmitters block the messages or signals from being passed along. Modulatory neurotransmitters influence the effects of the other neurotransmitters. They adjust how cells communicate at the synapse, which affects a large number of neurons at the same time (Neurotransmitters: What They Are, Functions & Types, 2022). Scientists believe that there are at least 100 types of neurotransmitters and suspect that there are many more. Some of the most important neurotransmitters include acetylcholine, norepinephrine, dopamine, gamma-aminobutyric acid (GABA), glutamate, serotonin, and histamine (Vasković, 2023). Some neurotransmitters are also involved in memory. Glutamate is the most common neurotransmitter involved in memory. Glutamate is an excitatory neurotransmitter and has been linked to spatial learning and hippocampus-dependent memory processes (Menard et al., 2012). Other neurotransmitters such as GABA, dopamine, and serotonin also seem to be involved in memory formation (Meneses, 2013). Overall, neurotransmitters and the MTL are vital to the body for proper memory formation and storage.
Encoding, Consolidation, Retrieval
The storage of learned information in the brain is referred to as memory (Guskjolen and Cembrowski, 2023). The neurons that are understood to be “mediating” the human memory are called “engrams”. The idea of engrams was first theorised by Richard Semon on the basis that changes in specific brain cells contain information and are reactivated upon retrieval (Josselyn and Tonegawa, 2020). Engrams can be thought of as the neural substrate for storing and recalling memory (Josselyn and Tonegawa, 2020).
There are two engram cell-specific properties (Tonegawa et al., 2015). Engrams have an increased dendritic spine density and show higher synaptic strength when compared to non-engram cells. In order to first store a lasting memory, there first must be some plasticity of engram cell structure and/or physiology. Protein synthesis is crucial for memory consolidation, as evidenced by a study involving animals administered with the protein synthesis inhibitor anisomycin. The results showed that the animals experienced retrograde amnesia one day later. However, when the anisomycin was administered one day after training, the engram cell was not affected. This tells us that memory consolidation also requires target training experience.
It is proposed that the increased engram cell-specific synaptic strength is vital for the retrieval of specific memory engrams. It is important to note that the information content of the memory itself is encoded in a pattern of engram cell ensemble connectivity. A functional memory necessitates multiple nodes on an engram cell ensemble circuit. Nodes are essentially points where two or more engrams meet within a circuit of engram cells. This statement is proven true because studies have shown that individual fear memories require engram cells from multiple brain regions (Guskjolen and Cembrowski, 2023).
Implicit vs Explicit Memory
The brain retains different kinds of memory. It can be divided into long-term memory and short-term memory. Long-term memory can be further divided into implicit and explicit memory. Explicit memories are conscious memories that humans intentionally recall (Yang and Li, 2012). It can be separated into two main categories, which are episodic and semantic memories. Episodic memories are also known as autobiographical memories; they refer to events that happened to a person. However, semantic memories are general knowledge of the world. Implicit memories are subconscious memories that can be separated into two main categories , which are procedural and priming memories (Yang and Li, 2012). Procedural memories are involved in learning new motor skills, such as riding a bicycle. Priming memories are sensitive to stimuli in the environment, which prompts them to recall certain information subconsciously (Squire and Dede, 2015). An example would be a person being primed with the colour blue is more likely to recall blue objects, such as the sky and the sea. Implicit and explicit memory are supported by different distinct brain networks. Explicit memory relies on the hippocampus (the temporal-parietal cortex in the brain), while the cortical-subcortical circuit (specifically the basal ganglia and the frontal cortex) is involved in implicit memory (Yang and Li, 2012). According to research, individual perception style influences implicit memory. A person’s sensory difference will affect subconscious processing more than conscious processing. This explains why a collective memory can be constructed among a group of people, such as history, whereas explicit memories are not influenced by an individual perception style (Hine and Tsushima, 2018). Furthermore, a study has been conducted to show that implicit memory for repeated search displays was significantly better after sleeping than a rest interval. In contrast, explicit learning did not benefit from a nap. Explicit memory for repeated search display improved the same after a rest interval and sleeping (Geyer et al., 2013). Both explicit and implicit memory declines with age. It is noted by Ward et al. (2020) that there is an increase in explicit memory up to 25 to 30 years old before the gradual decrease. However, results show that implicit memory has a smaller decline than explicit memory. This can be due to differences in task variety, as implicit tasks are more diverse than explicit tasks, making it more difficult to statistically detect the effects.
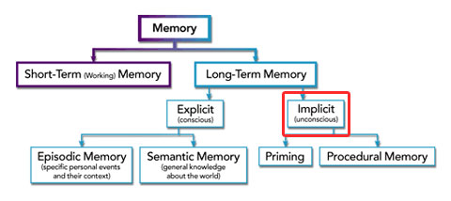
Figure 1: A flow diagram to show the difference between the short-term and long-term memory. The long-term memory consists of the explicit and implicit memory (DynamicBrain).
Synaptic Pruning and Neuroplasticity
At birth, a baby’s brain consists of 100 billion neurons and may be connected through over 100 trillion synaptic connections in the course of our life (Ackerman, S., 1992). Each neuron can be linked to more than 1000 other neurons, creating incredibly intricate neural circuits (Gulati A., 2015). Eventually, some synapses that are no longer in use will disappear while those that are still frequently used will remain. This was comprehended by the demonstration of computational models in which the brain’s learning process works most effectively when synaptic connections are first overproduced and then pruned back over time (Navlakha et al., 2015). In 1979, a paediatric neurologist, Peter Huttenlocher, discovered that the human brain starts its development in the early embryonic stage and reaches its maximum number of synaptic connections in early childhood, peaking at 1 to 2 years of age, at about 50% above adult levels (Huttenlocher, P., 1979). This number of synaptic connections rapidly declines during adolescence, stabilises in adulthood, and may potentially decline later in life. Psychiatrist, Irwin Feinberg, later defined this reduction as synaptic “pruning” (Feinberg, I., 1982).
Synaptic pruning is an activity-driven process, which abides by the “use it or lose it” principle, causing the synaptic region on the more active branches. The process frequently gathers the most recent or new information to be enlarged and protected, while being reduced or eliminated on the rarely active branches (Shors et al., 2012). Immune molecules called complement proteins, and the brain’s supportive immune cells, microglia, aid this act. Together, complement proteins will act as a chemoattractant and opsonize pathogens, while microglia engulf the complement proteins’ tagged pathogens, including unneeded synapses that are in low activity (Sakai, J., 2020). On the other hand, they can also stimulate active synapses and make their connections stronger. By deciding whether or not to keep a related memory and how tightly connected it should be to the necessary memory, synaptic pruning improves the efficiency of neural transmissions. This provides a more relevant substrate to remember new information so they can be recalled easily.
Synaptic pruning is part of neuroplasticity. As our nervous system undergoes functional or structural changes in response to intrinsic or extrinsic stimuli, our brain adapts to these experiences and rewires by reorganising its structure, functions, or connections throughout our life span (Mateos-Aparicio, P. and Rodríguez-Moreno, A., 2019). This process enables the brain to collect memories. It involves the formation and elimination of synapses as well as the modification of the strength of mature synaptic connections that carry our memories, whether as a result of brain damage or as a result of learning, experience, and memory creation (Cherry, K., 2020). Depending on the intensity and how often the stimulation is repeated, changes in synaptic strength can be temporary or long-lasting. (Kennedy MB, 2013). Fortunately, our brain is still able to gain new memories throughout our lifetime as neuroplasticity of the brain is not limited by age. But, it is much easier and more effective to influence a baby’s developing brain architecture than to rewire parts of its circuitry in adulthood (Center on the Developing Child, 2007). In response to learning new information, the brain creates new neurons and establishes new connections between them while eliminating those that are no longer used, to create an efficient pathway for repeating the learned behaviours.
Overall, neuroplasticity, which performs synaptic pruning, aids in the efficient transmission of information and organisation of our memories. As the foundation of learning and performing, a necessary role in the formation of refined neural circuits, neuroplasticity is an essential factor for optimal brain development.
MEMORY DERAILED: THE TYPES AND MECHANISMS OF AMNESIA
Retrograde and Anterograde Amnesia, and the Lifespan Retrieval Curve
Retrograde amnesia (RA) and anterograde amnesia (AA) are two distinct types of amnesia, and have often been found to occur simultaneously (Smith et al., 2013). Even though, in most cases, both these types of amnesia arise from damage to brain structures forming the limbic system, like the hippocampus , they differ in several aspects such as temporal profiles and affected memory systems (Mayo Clinic, 2022).
RA refers to the loss of memory for information encoded and consolidated before the onset of amnesia (Reed and Squire, 1998). The extent of RA varies significantly, with loss of memory ranging from a year to decades prior to the onset of amnesia. Contrastingly, individuals with AA have intact memories of prior events, but have an impaired capacity for encoding and consolidating new information after the onset of amnesia (Smith et al., 2013).
Depending on its severity and the brain regions affected, RA can be temporally graded or flat. Temporally Graded Retrograde Amnesia (TGRA) refers to a phenomenon where recently acquired information is more severely impaired than more remotely acquired information (Clark et al., 2002). Alternatively, Flat RA consists of a uniform memory deficit for all pre-amnesic information, regardless of the time period (Brown, 2002). This difference between the types of RA suggests the presence of a long-term encoding and consolidation process. On the other hand, AA typically presents a consistent inability to form new memories, regardless of temporal distance.
Individuals with RA have impaired explicit memory, but are still able to form new memories due to the retention of procedural memory. A study by Epp et al. (2008) also found that RA severity for visual memory is negatively correlated with spared hippocampal tissue volume. On the other hand, in individuals with AA, episodic, semantic, or both types of declarative memory can be impaired. However, procedural memories are often preserved in these individuals, as seen in patient HM.
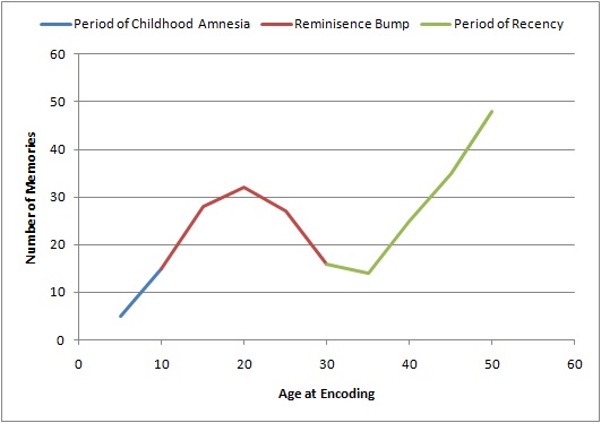
Figure 2: A Graph showing the Lifespan Retrieval Curve. [Taken from (Wikipedia Contributors, 2019).]
Since RA and AA represent distinct types of memory impairment, understanding the dynamics of memory retrieval over a lifetime can help shed light on them. This can be interpreted from the Lifespan Retrieval Curve, consisting of three parts: the period of childhood amnesia (from birth to 5 years), the reminiscence bump (10-30 years), and the period of recency (from the present dating back to the reminiscence bump).
The period of childhood amnesia has been attributed to either the underdevelopment of the infant’s brain, which would inhibit memory consolidation, or simply to deficits in memory retrieval. However, even though the actual memories from this time period are inaccessible to an adult, events such as neglect can still impact adult behaviour and even predispose individuals to various psychopathologies (Alberini and Travaglia, 2017). The reminiscence bump and period of recency refer to the phenomenon whereby older adults have a greater recollection of events from their adolescence and early adulthood. There are several theories for why this happens, including the fact that most significant life events, such as graduation and marriages, happen during this period.
The reminiscence bump is especially relevant because according to Conway (2005), the Self-Memory System (SMS) is thought to be bidirectional -. our memories provide context for ourselves and we control memory retrieval. As in the graph, autobiographical memories are not evenly distributed across our lifetime but appear to peak during the reminiscence bump. Rathbone, Moulin and Conway (2008) argued that when a new self-image is formed, it remains accessible even years later due to its association with the encoding of memories that are relevant to that self. However, in a study conducted by Grilli and Verfaellie (2015), it was found that amnesic patients were able to provide fewer episodic memories to support their self-statements, such as their “I am” statements, as compared to the control. Due to the contrasting nature of these studies, further research is required to offer insight into understanding the relationship between memory and identity, and how the latter is affected by amnesia.
Does Amnesia affect implicit memory? Patient H.M.
Does amnesia affect implicit memory? This depends on the type of amnesia a person has. Implicit memory refers to the unconscious and autonomic memory, doing everyday tasks that we don’t have to think about such as riding a bike or brushing our teeth in the morning (Cleveland Clinic). If a person is diagnosed with amnesia, whether it is AA or another form of amnesia, it will not affect their implicit memory because they would already know how to brush their teeth, how to ride a bike, and how to walk. These are everyday tasks that are ingrained in the human brain.
Henry Gustav Molaison, better known as patient H.M., underwent brain surgery which required the removal of his hippocampus and amygdala in order to control his seizures. Patient H.M. is a famous case study for anterograde and retrograde amnesia and many psychologists refer to this study for evidence in many articles. As a result of his surgery, his seizures were reduced, however he was unable to form new memories or remember the prior 11 years of his life. Because of this, he lost the ability to remember new facts and faces. (Erine Heaning, 2023). However, Patient H.M. was also able to complete tasks that he never did before, such as improving his reverse-mirror drawing skills over time and remembering how to mow the lawn. This showed that his procedural memory is intact through practising the specific task he wanted to complete, and over time this improved his procedural memory (Erin Heaning, 2023). Researchers also found that he was able to remember his house and draw a floor plan of his new home. This showed that he was able to form a cognitive map of the spatial layout of his home.
“Supermemory” / Savant Syndrome
Often dubbed as superhuman calculators, individuals with the famed savant syndrome share many qualities related to memory and developmental behavioural issues. Whilst it could be described as a trade-off of autism for extremely specific skills, there is no denying that patients with the syndrome almost always gain strong memorization abilities. In contrast to other topics discussed, Savants seem to be amnesiacs in complete reverse. The formation of this can be traced to either an inborn condition or a sudden injury to a specific part of the brain (Lythgoe et al. 2005).
Acquired savants are usually people who are diagnosed with savant syndrome following an injury. Although the exact way in which the acquired savant’s memory is so much stronger is still unofficial, some studies explain this better. The neocortex is a portion of the brain in the parietal cortex which covers one’s cognition and episodic memory abilities, in specific the episodic memory ability. The anatomic substrate for savant through conducted studies shows an enhancement in function for the neocortex in the parietal cortex, whilst simultaneously demonstrating loss of function in the left temporal lobe (Hou et Al., 2000). The left temporal lobe is responsible for the understanding of language and spatial awareness. Damage to the temporal lobe may result in autistic symptoms that can occur in patients with the syndrome, as the loss of language makes communication harder and emotions harder to grasp. Another theory relates to older patients and frontotemporal dementia. Frontotemporal dementia (FTD), as the name implies, affects the frontal and temporal lobes. As older patients’ temporal lobe slowly become damaged from memory loss due to FTD, their savant tendencies increase at a prodigious rate (Miller et al. 1998; Miller et al., 2000). The aforementioned two studies share similar results on injuries to the temporal lobe, the left portion in focus. This brings forth the supposed right-sided brain compensation, resulting in the uptake of mechanical and savant skill sets that could be described as super memory (Kapur, 1996).
There is also inborn savant syndrome. This refers to the syndrome not coming from an injury or disease to the brain after infancy, but one that a newborn has had since birth. Studies have shown that the most likely introduction of this stems from chromosomal disorders, brain malformation and prenatal disease (Treffert, 2006). The difference between acquired and inborn typically only results in an inborn having more time to facilitate their ability leading to it becoming stronger but is otherwise very similar. Dually noted is that the condition, similar to autism, is far more likely in boys than in girls, with a ratio of six to one (Geschwind and Galburda, 1987).
Savants have been widely popularised by media including movies, and they offer us a unique and intriguing view of the details of amnesia and memory loss. Savants cannot control what they choose to remember, but being a cab driver and remembering thousands of streets may strengthen your memory.
“The London Cab Driver”
London cab drivers are required to learn every road and route in London, known as “being on The Knowledge”.
A study conducted on London cab drivers found that individual brain plasticity is capable of making changes for the sake of a person’s career or learning a new skill. Magnetic resonance imaging (MRI) scans have shown that cab drivers’ brains, compared to non-cab drivers who were similar in age, education, and intelligence level, had more dense grey matter. This indicates that they undergo a ballooning hippocampus stage, in which their hippocampus starts growing new neurons and making stronger connections due to the ongoing practice. Over the four years of studying, their brain structure changes and they start building new connections and neurons that help them retrieve the information needed to pass every route in London with ease.
Another study used voxel-based morphometry (VBM), which is used to measure focal brain volume, and concluded that the amount of grey matter in an individual’s brain is distributed according to their need. In the case of cab drivers, it was shown that they have greater grey matter in their posterior hippocampus, which is responsible for the retrieval of memory and information. Non-cab drivers had more grey matter in their anterior hippocampus, which is responsible for memory encoding. This shows the plasticity of the brain and its ability to change according to a person’s needs.
The glial cells, which are responsible for supporting and protecting neurons, also aid in increasing the volume of the hippocampus. However, as a trade-off, cab drivers’ frontal lobes eventually shrink, which is responsible for making connections that help individuals access their sensory and planning functions. Despite becoming very good at a certain skill, this can cause them to become worse at others. This does not reflect what a healthy brain looks like, as the frontal lobe is usually the largest in a healthy brain. Shrinkage can cause numerous malfunctions such as memory loss. It was further concluded that after retirement, cab drivers’ hippocampus starts shrinking back to normal as it is not in use anymore. This is similar to when a person who regularly visits the gym and lifts weights then suddenly stops, all the muscle gain will eventually go back to normal.
In summary, while people have different abilities to consume knowledge, this study has shown that brain plasticity can change with constant practice. This means that anyone can improve their ability to retrieve knowledge. The study also indicates that a person’s ability to learn and retain knowledge can change depending on their career or the acquisition of a new skill.
DISCUSSION
This research paper investigated the science of memory formation and the types and mechanisms of amnesia. A major finding is that since different brain networks are responsible for implicit and explicit memory, factors affecting memory may not be the same.
An unexpected finding of implicit and explicit memory is that napping does not benefit explicit memory as the hippocampus, which supports explicit memory, is known as a core structure for sleep-dependent memory consolidation. The possible alternative explanation of this result is that explicit memory requires active interaction with the subject to be learned. If a person does not have enough time to engage with the material, there may be limitations in the memory consolidation process during the nap. Additionally, other factors, such as daytime sleep periods and intervals between learning and testing, may have affected the results of this research.
A study by paediatric neurologist, Peter Huttenlocher, showed that the human brain starts its development from the early embryonic stage and increases to its maximum number of synaptic connections in early childhood. This peaks at about 50% above adult levels between ages 1 to 2, and then decreases rapidly during adolescence, stabilises in maturity, and continues with a potential decline in synaptic density later in life. This process can be demonstrated by computational models which show that the brain’s learning process works most effectively when synaptic connections are initially overproduced and then gradually pruned back over time (Navlakha et al., 2015).
With this paper, the main focus was on amnesia and its different types. With the currently available knowledge of amnesia, this research paper discusses where in the brain amnesia is affected (MTL), the two important memory types (implicit/explicit memory), and synaptic pruning. This paper was able to draw conclusions about the relationships between neurotransmitters and memory and how some neurotransmitters were involved in memory formation. Also the processes of memory formation, which are encoding, consolidation, and retrieval, and how important they are to understanding retrograde and anterograde amnesia.
This study has introduced us to how memories are stored, and retrieved, and the main causes of memory loss, such as amnesia. However, it’s important to acknowledge that there are limitations to this research. The methodology was constrained by external factors, such as tension, anxiety, and environmental factors, which were not taken into account during the research process. Another limitation that should be considered is the health of the patient’s memory engram. The brain is a complex organ, and it requires a healthy memory engram to be able to retain knowledge. Damage to the engram may be the cause of apparent amnesia cases. Despite not exploring every aspect of the brain, the research still provides valuable insights into the nature of memory and how the brain’s plasticity is capable of gaining and retrieving new knowledge, even in patients with amnesia like patient H.M.
While examining patients with amnesia through observation and psychometric testing has been a key factor in helping researchers understand more about how memory is processed in the brain, much more sophisticated research is required to implement treatments. On a molecular and cellular level, research delving into changes in gene expression, neurotransmitter levels, and protein synthesis during amnesia can help identify targets for drug therapies. Additionally, neurostimulation approaches, like in the study that used light to revive lost memories in mice, can be further explored to provide a viable therapeutic option. Finally, another scope of further research is the impact of amnesia on identity. While this topic has been touched upon in a few studies like the two discussed in this paper, the majority of research is murky and has conflicting results. Further research in this field can aid in long-term patient care by at least improving quality of life and coping strategies, if not forging a way towards complete treatment of amnesia.
CONCLUSION
Memory is a gargantuan field, which has many subsections to it that have been explored in this paper. In the case of the MTL, it has been known to control functions of episodic memory and learning. The processes of memory function in the MTL include encoding, retrieval and consolidation. Neurotransmitters are chemical messengers that help the nervous system with communication. With the memory stored, it can be divided further into long-term memory which is categorised into explicit (conscious) and implicit (subconscious), each supported by different brain networks. Both decline with age, with explicit memory declining more. Synaptic pruning is an activity-driven process that depends on microglia to engulf and eliminate excess synapses in the human brain.
Despite these gains, the efficiency from pruning is sent into turmoil upon injuries or diseases to the brain that affect knowledge of the past or in other cases disable your consolidation of current information. Thankfully, skillsets are not affected that much by memory loss, which can be in part asserted by the contributions of patient HM to neuroscience. Furthermore, whilst memory generally worsens with age, in the case of an unlikely over-function in the left temporal lobe, the opposite occurs. Known as savant syndrome, it can be described as a trade-off of extreme memory but no control. Lastly, although in less effect, those who wish to only reinforce specific memory purposefully also improve the recollection of their brain as pointed out by the London Cab drivers.
The goal of this study has been to link parts of Amnesia together to inform. In retrospect, whilst we are not fully aware of what causes Amnesia in general, over time continuing research and carrying over the ideas provided will keep your memory in shape.
References
Ackerman, S. (1992). The Development and Shaping of the Brain. [online] www.ncbi.nlm.nih.gov. National Academies Press (US). Available at: https://www.ncbi.nlm.nih.gov/books/NBK234146/.
Alberini, C.M. and Travaglia, A. (2017). Infantile Amnesia: A Critical Period of Learning to Learn and Remember. The Journal of Neuroscience, [online] 37(24), pp.5783–5795. doi:https://doi.org/10.1523/jneurosci.0324-17.2017.
Anon (2017). Center on the Developing Child at Harvard University. [online] Center on the Developing Child at Harvard University. Available at: https://www.developingchild.harvard.edu.
Balder, J. (n.d.). What the Black Cab Drivers of London Taught Us About Learning and Ability. [online] www.wiley.com. Available at: https://www.wiley.com/learn/jossey-bass/what-the-black-cab-drivers-of-london-taught-us-about-learning-and-ability.html#:~:text=This%20confirmed%20the%20scientists%27%20conclusion [Accessed 20 Jul. 2023].
Bayley, P.J., Hopkins, R.O. and Squire, L.R. (2006). The Fate of Old Memories after Medial Temporal Lobe Damage. Journal of Neuroscience, [online] 26(51), pp.13311–13317. doi:https://doi.org/10.1523/jneurosci.4262-06.2006.
Brown, A.S. (2002). Consolidation theory and retrograde amnesia in humans. Psychonomic Bulletin & Review, [online] 9(3), pp.403–425. doi:https://doi.org/10.3758/bf03196300.
Cherry, K. (2022a). What is Neuroplasticity? [online] Verywell Mind. Available at: https://www.verywellmind.com/what-is-brain-plasticity-2794886.
Cherry, K. (2022b). What’s the Difference Between Implicit and Explicit Memory? [online] Verywell Mind. Available at: http://www.verywellmind.com/implicit-and-explicit-memory-2795346 [Accessed 29 Jul. 2023].
Clark, R.E., Broadbent, N.J., Zola, S.M. and Squire, L.R. (2002). Anterograde Amnesia and Temporally Graded Retrograde Amnesia for a Nonspatial Memory Task after Lesions of Hippocampus and Subiculum. The Journal of Neuroscience, [online] 22(11), pp.4663–4669. doi:https://doi.org/10.1523/jneurosci.22-11-04663.2002.
Cleveland Clinic (2022). Neurotransmitters: What They Are, Functions & Types. [online] Cleveland Clinic. Available at: https://my.clevelandclinic.org/health/articles/22513-neurotransmitters [Accessed 22 Jul. 2023].
Cleveland Clinic. (2020). Dissociative Amnesia: Symptoms, Causes, Management & Treatment. [online] Available at: https://my.clevelandclinic.org/health/diseases/9789-dissociative-amnesia#:~:text=There%20may%20be%20a%20genetic.
Conway, M.A. (2005). Memory and the self. Journal of Memory and Language, [online] 53(4), pp.594–628. doi:https://doi.org/10.1016/j.jml.2005.08.005.
Culture. (2013). The Bigger Brains of London Taxi Drivers. [online] Available at: https://www.nationalgeographic.com/culture/article/the-bigger-brains-of-london-taxi-drivers.
Cutsuridis, V. and Yoshida, M. (2017). Editorial: Memory Processes in Medial Temporal Lobe: Experimental, Theoretical and Computational Approaches. Frontiers in Systems Neuroscience, 11. doi:https://doi.org/10.3389/fnsys.2017.00019.
Das, S.R., Pluta, J., Mancuso, L., Kliot, D., Yushkevich, P.A. and Wolk, D.A. (2015). Anterior and posterior MTL networks in aging and MCI. Neurobiology of Aging, 36, pp.S141-S150.e1. doi:https://doi.org/10.1016/j.neurobiolaging.2014.03.041.
Epp, J., Keith, J.R., Spanswick, S.C., Stone, J.C., Prusky, G.T. and Sutherland, R.J. (2008). Retrograde amnesia for visual memories after hippocampal damage in rats. Learning & Memory, [online] 15(4), pp.214–221. doi:https://doi.org/10.1101/lm.788008.
Faust, T.E., Gunner, G. and Schafer, D.P. (2021). Mechanisms governing activity-dependent synaptic pruning in the developing mammalian CNS. Nature Reviews Neuroscience, 22(11), pp.657–673. doi:https://doi.org/10.1038/s41583-021-00507-y.
Feinberg, I. (1982). Schizophrenia: Caused by a fault in programmed synaptic elimination during adolescence? Journal of Psychiatric Research, 17(4), pp.319–334. doi:https://doi.org/10.1016/0022-3956(82)90038-3.
Ferris Jabr (2011). Cache Cab: Taxi Drivers’ Brains Grow to Navigate London’s Streets. [online] Scientific American. Available at: https://www.scientificamerican.com/article/london-taxi-memory/.
Foerde, K. (2010). Implicit Learning and Memory: Psychological and Neural Aspects. [online] ScienceDirect. Available at: https://www.sciencedirect.com/science/article/abs/pii/B9780080453965001391 [Accessed 29 Jul. 2023].
Geschwind, N. (1985). Cerebral Lateralization. Archives of Neurology, 42(5), p.428. doi:https://doi.org/10.1001/archneur.1985.04060050026008.
Geyer, T., Mueller, H.J., Assumpcao, L. and Gais, S. (2013). Sleep-Effects on Implicit and Explicit Memory in Repeated Visual Search. PLoS ONE, 8(8), p.e69953. doi:https://doi.org/10.1371/journal.pone.0069953.
Grilli, M.D. and Verfaellie, M. (2015). Supporting the self-concept with memory: insight from amnesia. Social Cognitive and Affective Neuroscience, [online] 10(12), pp.1684–1692. doi:https://doi.org/10.1093/scan/nsv056.
Gulati, A. (2015). Understanding neurogenesis in the adult human brain. Indian Journal of Pharmacology, [online] 47(6), p.583. doi:https://doi.org/10.4103/0253-7613.169598.
Guskjolen, A. and Cembrowski, M.S. (2023). Engram neurons: Encoding, consolidation, retrieval, and forgetting of memory. Molecular Psychiatry, [online] pp.1–13. doi:10.1038/s41380-023-02137-5.
Hernandez, P.J. and Abel, T. (2008). The role of protein synthesis in memory consolidation: Progress amid decades of debate. Neurobiology of Learning and Memory, [online] 89(3), pp.293–311. doi:https://doi.org/10.1016/j.nlm.2007.09.010.
Hine, K. and Tsushima, Y. (2018). Not explicit but implicit memory is influenced by individual perception style. PLOS ONE, 13(1), p.e0191654. doi:https://doi.org/10.1371/journal.pone.0191654.
Hou, C., Miller, B.L., Cummings, J.L., Goldberg, M., Mychack, P., Bottino, V. and Benson, D.F. (2000). Autistic savants. [correction of artistic]. Neuropsychiatry, neuropsychology, and behavioral neurology, [online] 13(1), pp.29–38. Available at: https://europepmc.org/article/med/10645734 [Accessed 29 Jul. 2023].
Josselyn, S.A. and Tonegawa, S. (2020). Memory engrams: Recalling the past and imagining the future. Science, [online] 367(6473). doi:https://doi.org/10.1126/science.aaw4325.
Kapur, N. (1996). Paradoxical functional facilitation in brain-behaviour research. Brain, 119(5), pp.1775–1790. doi:https://doi.org/10.1093/brain/119.5.1775.
Kendra Cherry (2019). What’s the Difference Between Implicit and Explicit Long-Term Memory? [online] Verywell Mind. Available at: https://www.verywellmind.com/implicit-and-explicit-memory-2795346.
Kennedy, M.B. (2016). Synaptic Signaling in Learning and Memory. Cold Spring Harbor Perspectives in Biology, [online] 8(2). doi:https://doi.org/10.1101/cshperspect.a016824.
Kumar, M. (2022). Factors Affecting Memory Recall. [online] www.tutorialspoint.com. Available at: https://www.tutorialspoint.com/factors-affecting-memory-recall#:~:text=Emotion%20and%20external%20factors%20might.
Langer, K.G. (2019). Early History of Amnesia. Frontiers of neurology and neuroscience, [online] 44, pp.64–74. doi:https://doi.org/10.1159/000494953.
Lythgoe, M.F.X., Pollak, T.A., Kalmus, M., de Haan, M. and Chong, W.K. (2005). Obsessive, prolific artistic output following subarachnoid hemorrhage. Neurology, [online] 64(2), pp.397–398. doi:https://doi.org/10.1212/01.WNL.0000150526.09499.3E.
Maguire, E.A., Gadian, D.G., Johnsrude, I.S., Good, C.D., Ashburner, J., Frackowiak, R.S.J. and Frith, C.D. (2000). Navigation-related structural change in the hippocampi of taxi drivers. Proceedings of the National Academy of Sciences, [online] 97(8), pp.4398–4403. doi:https://doi.org/10.1073/pnas.070039597.
Mateos-Aparicio, P. and Rodríguez-Moreno, A. (2019). The impact of studying Brain Plasticity. Frontiers in Cellular Neuroscience, [online] 13(66). doi:https://doi.org/10.3389/fncel.2019.00066.
Mayo Clinic (2022a). Amnesia – Diagnosis and treatment – Mayo Clinic. [online] www.mayoclinic.org. Available at: https://www.mayoclinic.org/diseases-conditions/amnesia/diagnosis-treatment/drc-20353366#:~:text=Research%20may%20one%20day%20lead [Accessed 14 Jul. 2023].
Mayo Clinic. (2022b). Amnesia – Symptoms and causes. [online] Available at: https://www.mayoclinic.org/diseases-conditions/amnesia/symptoms-causes/syc-20353360#:~:text=Amnesia%20can%20result%20from%20damage [Accessed 21 Jul. 2023].
Ménard, C. and Quirion, R. (2012). Group 1 Metabotropic Glutamate Receptor Function and Its Regulation of Learning and Memory in the Aging Brain. Frontiers in Pharmacology, 3. doi:https://doi.org/10.3389/fphar.2012.00182.
Miller, B.L., Boone, K., Cummings, J.L., Read, S.L. and Mishkin, F. (2000). Functional correlates of musical and visual ability in frontotemporal dementia. British Journal of Psychiatry, 176(5), pp.458–463. doi:https://doi.org/10.1192/bjp.176.5.458.
Miller, B.L., Cummings, J., Mishkin, F., Boone, K., Prince, F., Ponton, M. and Cotman, C. (1998). Emergence of artistic talent in frontotemporal dementia. Neurology, 51(4), pp.978–982. doi:https://doi.org/10.1212/wnl.51.4.978.
Navlakha, S., Barth, A. and Bar-Joseph, Z. (2015). Decreasing-Rate Pruning Optimizes the Construction of Efficient and Robust Distributed Networks. PLoS Comput Biol, [online] 11(7), p.1004347. Available at: https://pdfs.semanticscholar.org/5b12/8e78d75cd15dc2ea732a43d7d75ff98486ac.pdf?_gl=1 [Accessed 22 Jul. 2023].
Nurnberg, E. (2019). How the brain stores memories › Friedrich-Alexander-Universität Erlangen-Nürnberg. [online] FAU. Available at: https://www.fau.eu/2019/11/12/news/research/how-the-brain-stores-memories/#:~:text=When%20a%20memory%20is%20created.
Pappas, S. (2022). How does the brain store memories? [online] livescience.com. Available at: https://www.livescience.com/how-the-brain-stores-memories [Accessed 27 Sep. 2022].
Peter R., H. (1979). Synaptic density in human frontal cortex — Developmental changes and effects of aging. Brain Research, 163(2), pp.195–205. doi:https://doi.org/10.1016/0006-8993(79)90349-4.
Rathbone, C.J., Moulin, C.J.A. and Conway, M.A. (2008). Self-centered memories: The reminiscence bump and the self. Memory & Cognition, [online] 36(8), pp.1403–1414. doi:https://doi.org/10.3758/mc.36.8.1403.
Reed, J.M. and Squire, L.R. (1998). Retrograde Amnesia for Facts and Events: Findings from Four New Cases. The Journal of Neuroscience, [online] 18(10), pp.3943–3954. doi:https://doi.org/10.1523/jneurosci.18-10-03943.1998.
Sakai, J. (2020). Core Concept: How synaptic pruning shapes neural wiring during development and, possibly, in disease. Proceedings of the National Academy of Sciences, 117(28), pp.16096–16099. doi:https://doi.org/10.1073/pnas.2010281117.
Schacter, D.L. (1987). Implicit expressions of memory in organic amnesia: learning of new facts and associations. Human Neurobiology, [online] 6(2), pp.107–118. Available at: https://pubmed.ncbi.nlm.nih.gov/3624022/.
Scoville, W.B. and Milner, B. (1957). LOSS OF RECENT MEMORY AFTER BILATERAL HIPPOCAMPAL LESIONS. Journal of Neurology, Neurosurgery, and Psychiatry, [online] 20(1), pp.11–21. Available at: https://www.ncbi.nlm.nih.gov/pmc/articles/PMC497229/.
Shors, T.J., Anderson, M.L., Curlik, D.M. and Nokia, M.S. (2012). Use it or lose it: How neurogenesis keeps the brain fit for learning. Behavioural Brain Research, 227(2), pp.450–458. doi:https://doi.org/10.1016/j.bbr.2011.04.023.
Smith, C.N., Frascino, J.C., Hopkins, R.O. and Squire, L.R. (2013). The nature of anterograde and retrograde memory impairment after damage to the medial temporal lobe. Neuropsychologia, [online] 51(13), pp.2709–2714. doi:https://doi.org/10.1016/j.neuropsychologia.2013.09.015.
Squire, L.R. (2009). The Legacy of Patient H.M. for Neuroscience. Neuron, 61(1), pp.6–9. doi:https://doi.org/10.1016/j.neuron.2008.12.023.
Squire, L.R. and Dede, A.J.O. (2015). Conscious and Unconscious Memory Systems. Cold Spring Harbor Perspectives in Biology, [online] 7(3), p.a021667. doi:https://doi.org/10.1101/cshperspect.a021667.
Straube, B. (2012). An overview of the neuro-cognitive processes involved in the encoding, consolidation, and retrieval of true and false memories. Behavioral and Brain Functions, 8(1), p.35. doi:https://doi.org/10.1186/1744-9081-8-35.
Tonegawa, S., Pignatelli, M., Roy, D.S. and Ryan, T.J. (2015). Memory engram storage and retrieval. Current Opinion in Neurobiology, 35, pp.101–109. doi:https://doi.org/10.1016/j.conb.2015.07.009.
Treffert, D. (2006). Savant Syndrome | SSM Health Treffert Center. [online] Ssmhealth.com. Available at: https://www.ssmhealth.com/treffert-center/conditions-treatments/savant-syndrome.
Treffert, D.A. (2009). The savant syndrome: an extraordinary condition. A synopsis: past, present, future. Philosophical Transactions of the Royal Society B: Biological Sciences, [online] 364(1522), pp.1351–1357. doi:https://doi.org/10.1098/rstb.2008.0326.
Vasković, J. (2016). Neurotransmitters. [online] Kenhub. Available at: https://www.kenhub.com/en/library/anatomy/neurotransmitters [Accessed 28 Jul. 2023].
Ward, E.V., Berry, C.J., Shanks, D.R., Moller, P.L. and Czsiser, E. (2020). Aging Predicts Decline in Explicit and Implicit Memory: A Life-Span Study. Psychological Science, 31(9), pp.1071–1083. doi:https://doi.org/10.1177/0956797620927648.
Wikipedia Contributors (2019). Reminiscence bump. [online] Wikipedia. Available at: https://en.wikipedia.org/wiki/Reminiscence_bump [Accessed 29 Jul. 2023].
Yang, J. and Li, P. (2012). Brain Networks of Explicit and Implicit Learning. PLoS ONE, [online] 7(8), p.e42993. doi:https://doi.org/10.1371/journal.pone.0042993.