Supervised by: Faissal Sharif BSc, MSc, DPhil cand. (Oxon). Faissal obtained a BSc in Biomedical Sciences at Maastricht University with a semester abroad at the Chinese University of Hong Kong. As a visiting scholar at the University of California, Irvine, he investigated biomarkers for Alzheimer’s Disease. He completed his MSc in Translational Neuroscience at Imperial College London. Beyond his work on psychiatric disorders and mental health, Faissal has developed an interest in the connection between medicine and technology through working with Medtech and Digital Therapeutic startups and organizations such as the European Space Agency. Currently, he is completing his DPhil at Oxford University in Clinical Neuroscience, focusing on novel neurotechnology to fight mental disorders.
Introduction
This paper will provide an overview of cutting-edge research in Neurotechnology, including clinical findings on the therapeutic use of neuromodulation techniques such as Deep Brain Stimulation (DBS), and different methods of Non-invasive Brain Stimulation (NIBS), including Transcranial Magnetic Stimulation (TMS) and Transcranial Electric Stimulation (tES). These techniques fall under the field of Neuromodulation, a technology involving neural interfaces, including processes of inhibition, modulation and stimulation of the nervous system, to improve quality of life and restore health.
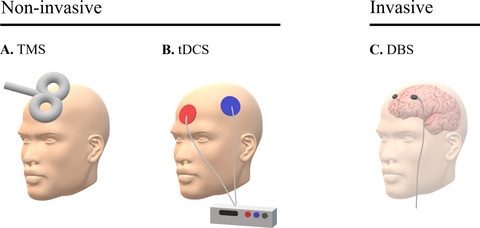
Figure A. Overview of different neuromodulation methods.
Adopted from Camacho-Conde et al., 2021.
Literature Review
Deep Brain Simulation (DBS)
DBS is an invasive technique that involves placing an electrode inside the brain at a subcortical level, as well as an implantable pulse generator (IPG), which is used to manage the intensity of the electrical currents to distinct parts of the brain (Fakhar et al., 2013). The IPG is a pacemaker, and it not only manages but also generates stimulation (Krauss et al., 2020). It is also possible for electrical impulses to affect certain brain cells and chemicals. This shows the possibility for DBS to have therapeutic properties for diseases like Tourette’s, psychiatric disorders, pain, and many more. For example, electric impulses are sent directly into the brain to help cure tremors and dystonia in Parkinson’s Disease (PD) (Aans.org, 2019; Mayo Clinic, 2018).
The surgery involves two steps. The first step involves taking an MRI of the brain so that the surgeon can visually inspect and identify in which sections of the brain the electrodes will need to be implanted. With the aid of a small drill, a small incision is created on the outer layers of the brain for wires to pass through. The second step involves implanting an IPG near the collarbone, placed under your skin.
History
The first clinical test with DBS was conducted in 1947, with the goal of treating the patient’s depression and anorexia. This first attempt was followed closely with high hopes for the final results. Unfortunately, the wire broke, which ended the experiment. Over the next few years, American and Canadian universities experimented with the procedure and the field progressed significantly. Unfortunately, in the 1980s, these advancements slowed for around 20 years (Krauss et al., 2020). It is only in more recent years that researchers have restarted making headway with this procedure (Montgomery & Gale, 2008). For example, patients no longer need to be awake during the second part of the surgery – they can be asleep, which helps reduce surgery-related stress.
DBS in Parkinson’s Disease (PD)
DBS of the subthalamic nucleus (STN–DBS) is a surgical treatment for PD that involves implanting electrodes in the STN to deliver electrical stimulation and improve motor symptoms. DBS of the globus pallidus internus (GPi) is another surgical treatment for PD that involves implanting electrodes in the GPi to deliver electrical stimulation to specific areas of the brain. The GPi and STN are both part of the basal ganglia, a group of structures in the brain that are involved in motor control. While DBS of the STN is typically used to treat the motor symptoms of PD, such as tremors and stiffness, DBS of the GPi may be more effective at reducing dyskinesias, which are involuntary movements that can occur as a side effect of medication for PD. The choice between DBS of the STN and GPi may depend on the individual patient and their specific symptoms and needs. STN-DBS has been proved to have a more positive impact on cardinal motor symptoms than gait and posture. These latter aspects are better protected and maintained with GPi DBS.
This subcortical intervention does come with some downsides, especially for patients with PD, where there is still a lot of room for progress in this growing field of research. One of the major pitfalls is the unrealistically high expectations of many patients on how the surgery will turn out. They often believe that their symptoms will fully or almost fully stop and that their life will dramatically improve (Rossi et al., 2018). However, this is not true in a lot of cases. Their post-surgery goals for their home, work and social lives are simply too high, and disappointment is inevitable. While DBS allows for a better quality of life (QoL) in its patients, speech disturbances are fairly common side effects for STN-DBS patients, despite being manageable with speech therapy or attenuated stimulation. More than one factor usually underlies the decline of a patient’s condition: these can range from a misplaced electrode, to the simple progression of the disease over time (Rossi et al., 2018). One important factor to take into account is that, even with these few negative factors, DBS does give PD patients a better QoL, and remains a beneficial procedure (Peng et al., 2018).
DBS in Epilepsy
In the case of epilepsy, DBS is used for reducing seizures, and commonly involves implantation of the electrode in the anterior thalamic nucleus (ATN) and/or hippocampus. Different locations in the brain can be targeted using DBS for different objectives and outcomes. Once it is decided that the patient is a candidate for the procedure, clinicians still need to decide which target would be most beneficial. Unfortunately, although ATN-DBS patients generally have a higher success rate, this is accompanied by a higher risk of depression following the surgery. Furthermore, general risks relating to the implantation of the electrode include soft tissue infections, as well as asymptomatic intracranial haemorrhaging (Sprengers et al., 2014). This, even if asymptomatic (patients not noticing), can lead to death if left untreated (Naidech, 2011).
DBS in Schizophrenia
DBS can also be utilised to help treat schizophrenia. Schizophrenia is a mental health disorder that affects how an individual views reality. For example, patients usually experience a myriad of hallucinations and delusions. Fortunately, DBS can make a big impact on these individuals. Areas of the brain that are associated with schizophrenia such as the ventral striatum and the subgenual anterior cingulate cortex are targeted to activate neurons and fix abnormal neural networks of the brain. Thus, DBS opens up another door to helping schizophrenic individuals lead normal lives (Fick, 2016).
DBS in Tourette’s syndrome
Tourette’s syndrome (TS) is a neuropsychiatric disorder typically developed in childhood whereby individuals have constant motor and vocal tics. DBS is a potential therapeutic option to treat people with TS. Similarly to how schizophrenia is approached with DBS treatments, in TS the electrodes will be implanted in targeted locations of the brain such as the centromedian thalamus, the internal globus pallidus, the external globus pallidus, and the anterior limb of the internal capsule. Furthermore, during DBS in TS, high-frequency stimulation (HF-DBS) is more frequently utilised especially on targets like the basal ganglia and the thalamus as they are responsible for tics. The HF-DBS increases ionic changes which in turn affect the subcortical targets. This will cause an increase in potassium concentrations which has an impact on the neurons, eventually restoring irregular circuits.
However, even after DBS treatment, the response has a general delay, thus neuroplasticity has been taken into account along with the HF-DBS method. As further approaches have been introduced, researchers have observed a causal relationship between a decrease in dopamine and a subsequent decrease in tics. In conclusion, further methods are still being introduced and tested but these are the major approaches that have yielded positive results (Casagrande et al., 2019).
Transcranial Electric Stimulation (tES)
Neuromodulation also entails an array of non-invasive techniques, whose therapeutic use for pain management (as an example) could prove highly beneficial due to its non-pharmaceutical nature, thus avoiding complications such as drug interactions (Chen et al., 2021). tES induces neuroplastic effects in the human cortex via non-invasive electrical stimulation resulting in a modification of the synaptic transmission (Terney et al., 2008). Transcranial direct current stimulation (tDCS), transcranial alternating current stimulation (tACS) and transcranial random noise stimulation (tRNS) are all forms of tES.
History
The act of applying a direct electric current to determine if brain functions are altered is a remarkably old practice, existing for over 200 years. Many basic experiments which tested the effects of a direct current on an area of interest in the brain date back to before the 19th century. In 1801, Giovanni Aldini used direct current stimulation to improve the mood of patients, proving that the technique affected brain function. Studies in the 1960s by D. J. Albert discovered that positive and negative stimulation had differential effects on cortical excitability, increasing the interest surrounding tDCS and encouraging more research which continues to this day.
Transcranial direct current stimulation (tDCS)
tDCS involves the use of electrodes attached to the head to produce a constant, low, direct electric current. This form of neuromodulation was developed to aid patients suffering from brain injuries, neuropsychiatric disorders, and more. Over time, this has seen increased evidence as a form of therapy for depression. tDCS has been observed to alter perceptual, cognitive and behavioural functions.
tDCS is portable and wearable when the electric current is being applied to the scalp. It involves a positive or negative current being delivered via electrodes to a specific area of the brain. The result is immediate alterations of brain function that may outlast the stimulation itself. The position of the anodes and cathodes, which produce the negative and positive currents respectively, are placed in such a way as to ensure the current flows to the desired region. tDCS is too weak to trigger an action potential in a neuron, but instead changes the pattern of any neurons already experiencing activity. Essentially, the brain is already active when focusing on something whether that be learning or engaging in any activity, and tDCS is simply boosting this activity. This attribute has proven to be potentially useful in treating neurological and psychiatric disorders such as depression.
While tDCS should theoretically result in depression improvement, the findings of studies have shown mixed results. One review involved clinical trials and a randomised, sham-controlled trial with 120 patients, all of whom across all trials were diagnosed with major depressive disorder (MDD). The goal was to analyse the effects of tDCS and see whether there was any improvement in the patients. Some trials involved daily tDCS sessions for the treatment of MDD. Two types of tDCS were used, active and sham (fake) stimulation, and while it was found that active tDCS was statistically superior, not enough evidence has been found to warrant immediate treatment sessions in daily clinical practices. Other methods such as pharmacotherapy and rTMS (repetitive transcranial magnetic stimulation) are still recommended above this technique (Shiozawa et al., 2014).
Transcranial alternating current stimulation (tACS)
Transcranial alternating current stimulation (tACS) is a non-invasive form of brain stimulation. Similar to tDCS an anodal electrode and cathodal electrode are attached to the head. Electrical current is then delivered to the scalp and flows from one electrode to the other. With tACS, oscillatory frequencies can also be altered in specific parts of the brain. Brain oscillations are rhythmic patterns of electrophysiological activity oscillating at differing frequencies. tACS aims to stimulate naturally occurring brain oscillations to treat various diseases, e.g. pain disorders and psychiatric disorders (Elyamany et al., 2020, Guleyupoglu et al., 2013). Considering the broad range of diseases caused by abnormal oscillatory frequencies, tACS can be a viable therapeutic technology for a variety of disorders and diseases (Elyamany et al., 2020).
The technique of tACS is very similar to tDCS. However, some other parameters which have to be considered in the application of tACS are the duration and number of stimulation sessions, as well as the frequency, amplitude, phase shape and timing of the alternating current. Also, computational models are used to optimise the position of the electrodes and other stimulation parameters (Elyamany et al., 2020).
Recent research studies have shown that tACS can be applied in the treatment of a broad range of diseases. One area of application is schizophrenia, a neurodevelopment spectrum disorder, which is characterised by specific alterations of brain oscillations (Farcas & Iftene, 2022, Elyamany et al., 2020). The application of tACS in patients with schizophrenia led partly to a decrease in schizophrenia-related symptoms. In particular, tACS treatment resulted in a decrease in auditory hallucinations and an improvement in cognitive abilities (Farcas & Iftene, 2022). Conducted applications of tACS varied on the one hand in the frequency of the alternating current – for example, theta frequency (4,5 and 6 Hz) tACS or alpha frequency (10 Hz) tACS can be used. On the other hand, they also varied in the number and position of electrodes and the number of sessions.
Another disease characterised by altered brain oscillations is depression (Elyamany et al., 2020). As many patients show treatment resistance to conventional treatments, tACS is a new, urgently needed option for the treatment of depression, as Haller et al. (2020) pointed out. The researchers reported a case series of patients suffering from major depression disorder who were treated with tACS at gamma (40 HZ) frequency. The severity of the patient’s depression decreased by up to 85% during the treatment (Haller et al., 2020).
Furthermore, PD is also characterised by abnormal brain oscillations. tACS can be used to modulate these oscillations to improve abnormal PD-related behaviour. Electroencephalograms (EEGs) of those patients were also recorded, with the intention of optimising the application of tACS. In this way, stimulation frequency could be set individually for every patient (Del Felice et al., 2019).
Transcranial random noise stimulation (tRNS)
tRNS is a special type of tACS that uses random frequencies and is characterised by the use of electrical currents that arbitrarily alternate between the two electrodes at high frequencies (Moret et al., 2018, Potok et al., 2021, Battaglini et al., 2020).
In 2008, Terney et al. (2008) published a study introducing tRNS as a new technique to enhance corticospinal excitability by applying weak tRNS for 10 min over the motor cortex. To record behavioural changes, a serial reaction time task was used as a standard paradigm to test implicit motor learning. The study showed that tRNS constitutes another painless and non-invasive technique to enhance the excitability of the cortex (Terney et al., 2008). As a relatively new yet popular approach, various recent studies focus on the application of different parameters.
One parameter that was analysed is the frequency of the tRNS. While it was shown by previous studies that high-frequency band stimulations are most effective in enhancing neural excitability, it was unclear whether this relates to the full bandwidth of the high-frequency band (Moret et al., 2019). Moret and colleagues were able to prove that the efficacy of tRNS is strictly related to the width of the selected frequency range suggesting that the medium sub-range of the high-frequency band should be used.
One more recent study deals with a comparison between anodal tDCS and high-frequency tRNS regarding the motor cortical excitability changes concerning the duration of the stimulation (Haeckert et al., 2020). This study found that motor cortical excitability was relatively stable for anodal tDCS and independent from the stimulation duration, whereas motor cortical excitability was considerably impacted by the duration factor when high frequency tRNS was used. The modulatory effects of tRNS have been probed in various studies with different motor, sensory and cognitive tasks to determine task dependency. For example, one study explored dissociated effects of tRNS. Jooss et al. (2019) were able to prove that neuromodulatory effects induced by tRNS are task-dependent by asking probands to perform an activating and an inhibitory motor task. Studies do not only focus on evidencing the online effects of tRNS on corticospinal excitability and neuroplasticity, but also to prove if and under which conditions, the stimulation results in long-term offline effects (Brevet-Aeby et al., 2019). Brevet-Aeby et al. (2019) were able to provide evidence that the sustainability of tRNS can be improved if repeated tRNS sessions are performed on the same day compared to single sessions.
Various studies test the efficacy of tRNS in the context of certain diseases and the respective therapy. Antal & Herrmann (2016) reviewed various interdisciplinary research studies regarding tACS and tRNS to analyse the related effects on brain oscillations and the possibilities of modulating cortical functions. tRNS can be considered promising to apply in the therapy of neurological and psychiatric disorders that are due to malfunctioning brain oscillations.
Transcranial Magnetic Stimulation (TMS)
TMS is a noninvasive brain stimulation technique which uses magnetic fields to regulate nerve cells, and helps prevent the need for invasive surgery. TMS helps regulate brain activity and operates by the transmission of a pulse of current through a coil or wire (Eldaief et al., 2020). This generates the magnetic fields which lead to brain activation. There are many different forms of TMS application, including single or paired pulses. In summary, TMS (including single and paired-pulse TMS) is used to investigate how the brain works, whereas repeating TMS (rTMS) is used to cause alterations in the brain’s activity that can endure beyond the stimulation period. Electromyography (EMG) evidence shows that a motor-evoked potential (MEP) is induced after non-invasive application of TMS of the motor cortex induces a twitch in the target muscle (Klomjai et al., 2020). Cognitive changes and facial discomfort are associated with TMS, as they can be induced by the stimulation (Eldaief et al., 2020).
History
Since 1985, electromagnetic induction has been used for transcranial stimulation. In Sheffield, UK, around that period, Barker and his coworkers created the first type of TMS . Additionally, in 1985, Barker provided scientific proof of the impact of magnetic stimulation on the motor cortex of the human brain (Noohi & Salari, 2016). rTMS was initially only used to diagnose neuromotor diseases. Several years prior, researchers hypothesised that using rTMS to target specific cortical regions would have antidepressant-like effects. Nonetheless, early on in the development of TMS, the influence of TMS on the prefrontal area was not well publicised. Currently, devices for repeating electromagnetic induction have been created into a structure that allows for the exact modification of many parameters, such as the capacity to increase or decrease the magnetic force on cortical areas, to treat a variety of psychological illnesses (Noohi & Salari, 2016).
TMS in obsessive compulsive disorder (OCD)
Evidence that TMS is significantly effective and reliable at easing symptoms is required before it can be given a clear clinical role in the treatment of OCD. The results of current clinical studies and the growing body of knowledge regarding the effects of TMS on brain networks are reassuring, but they also illustrate the need for more research into the use of TMS as a method of selectively normalising OCD brain network dynamics and easing associated symptoms (Cirillo et al., 2019). The precision and therapeutic efficacy of TMS therapies could be enhanced by the integration of neuroimaging, computational modelling, and behavioural regimens that are known to engage brain networks impacted by OCD (Cirillo et al., 2019). The effectiveness of this multimodal strategy has yet to be shown, and it has technical and implementation difficulties when used effectively in clinical settings. To determine whether OCD can join major depressive disorder as a TMS-treatable disorder, these practical, scientific, and technical challenges must be addressed (Cirillo et al., 2019). Patients with severe OCD who are refractory to medication or behavioural therapy may benefit from various add-on stimulation modalities. Therefore, low-frequency TMS over the supplementary motor region or the orbitofrontal cortex might be more effective. Targeting the STN or the ventral capsule produced the best results with DBS. In the treatment of OCD, cathodal tDCS may be superior to anodal (Rapinesi et al., 2019).
TMS in PTSD
The anxiety condition known as post-traumatic stress disorder (PTSD) is brought on by experiencing or witnessing incredibly upsetting or frightful situations. The ventromedial prefrontal cortex, hippocampus, and amygdala are three important brain regions that might change as a result of stress. The amygdalae are a pair of almond-shaped structures in the brain that are involved in emotional processing and decision-making. According to brain imaging studies, PTSD sufferers have an overactive amygdala and decreased brain activity in the ventromedial prefrontal cortex (vmPFC). It is thought that severe anxiety results from the vmPFC losing control of the amygdala. Brain activity in the hippocampus, which aids in separating the present from the past, decreases in PTSD patients. This indicates that it can be challenging for PTSD sufferers to distinguish between current circumstances and past trauma. Electromagnetic impulses sent by an electromagnetic coil are used in TMS therapy to stimulate the cells in the vmPFC. Additionally, this therapy also regulates the activities of PTSD-related brain cells in other areas of the brain, possibly restoring them to baseline. Bringing the brain’s equilibrium and stability back to normal reduces the symptoms of PTSD. High frequency TMS across the right dorsolateral prefrontal cortex has been shown to be a significant overall treatment effect for both PTSD and generalised anxiety disorder (Cirillo et al., 2019).
Evaluation
TMS has a major therapeutic benefit on individuals’ lives while helping regulate brain activity. This benefit has been found in brain damage cases and in disorders like depression, addiction, and anxiety (Zorzo et al., 2019). There are many advantages to TMS. For example, it yields a high success rate of 68% in depression, compared to only 37.5% for the medication (Spielberg, 2020). Since TMS is non-invasive, it is safe and has the best side-effect profile. There are also a few disadvantages of TMS like having to visit a clinic during every treatment day. Patients generally need to attend treatment sessions 5 days per week for a total of 6 weeks (Spielberg, 2020). TMS can also cause individuals to feel some sort of discomfort. During the process, patients could feel a knocking through the magnetic pulses on their scalp, which is uncomfortable for some (Spielberg, 2020). There is also a lack of standardisation for TMS meaning that there are many different methods that could take place during this process.
DISCUSSION
Although all the techniques mentioned above are effective and promising for further use in research, aspects like safety, efficiency, cost and availability have to be put into consideration. TMS and tES, for example, may be cost-efficient treatments for disorders such as depression in developing countries. Furthermore, they are tolerable, non-invasive techniques that patients may be more comfortable with. In contrast with the latter procedures, DBS is an invasive method that involves some high risks, such as impairment of certain cerebral capacities. While tES is generally easy to administer due to it being portable and wearable, sometimes the process of modifying the parameters individually for every patient to optimise the application can be very time-consuming, especially as it needs to be done for each patient. This is comparable to DBS since the electrode is inserted inside the brain, and once it is healed, patients can go about their normal daily activities. tES has few side effects and the side effects which have been reported are relatively minor and temporary, such as skin redness around the electrode area. More research is needed to determine any lasting effects but tES is still a very safe technique. Conversely, DBS can have a very wide range of side effects, depending on what disease it is treating, but also just having a wire and electrode in the brain presents a risk for intracranial haemorrhaging, as well as soft tissue infections. Compared to DBS, TMS is safe and has the best side-effect profile due to it being non-invasive. Due to the simplicity of the different tES techniques, it should be easy for patients to access, particularly in developing countries. As they are usually portable, the availability of these treatments should not be an issue should they be proven valid in clinical practice. However, this simplicity is not found in DBS, as it is an invasive procedure.
Conclusion
Overall, it is clear that neuromodulation has great potential in the treatment of a wide variety of diseases, both mental and physical, as the techniques evolve over the years.
There are many opportunities to further advance the knowledge of these techniques so that they may be able to help people suffering from all kinds of conditions. Further research is required to make neuromodulation treatments more known to the public. Even though there are some side effects, neuromodulation techniques offer people with the aforementioned disorders a chance to lead a normal life with less suffering when their condition would have held them back otherwise.
References
Antal, A., & Herrmann, C. S. (2016). Transcranial Alternating Current and Random Noise Stimulation: Possible Mechanisms. Neural Plasticity, 2016(3616807), 1–12. https://doi.org/10.1155/2016/3616807
Battaglini, L., Contemori, G., Penzo, S., & Maniglia, M. (2020). tRNS effects on visual contrast detection. Neuroscience Letters, 717(134696), 134696. https://doi.org/10.1016/j.neulet.2019.134696
Boes, A. D., Kelly, M. S., Trapp, N. T., Stern, A. P., Press, D. Z., & Pascual-Leone, A. (2018). Noninvasive Brain Stimulation: Challenges and Opportunities for a New Clinical Specialty. The Journal of Neuropsychiatry and Clinical Neurosciences, 30(3), 173–179. https://doi.org/10.1176/appi.neuropsych.17110262
Brevet-Aeby, C., Mondino, M., Poulet, E., & Brunelin, J. (2019). Three repeated sessions of transcranial random noise stimulation (tRNS) leads to long-term effects on reaction time in the Go/No Go task. Neurophysiologie Clinique, 49(1), 27–32. https://doi.org/10.1016/j.neucli.2018.10.066
Camacho-Conde, J. A., Gonzalez-Bermudez, M. del R., Carretero-Rey, M., & Khan, Z. U (2021). Brain stimulation: a therapeutic approach for the treatment of neurological disorders. CNS Neuroscience & Therapeutics, 28(1), 5–18. https://doi.org/10.1111/cns.13769
Casagrande, S. C. B., Cury, R. G., Alho, E. J. L., & Fonoff, E. T. (2019). Deep brain stimulation in Tourette’s syndrome: evidence to date. Neuropsychiatric Disease and Treatment, 15(https://pubmed.ncbi.nlm.nih.gov/31114210), 1061–1075. https://doi.org/10.2147/NDT.S139368
Chen, Y.X., Zhang, L.B., Lyu, X.J., & Hu, L. (2021). [Non-invasive electrical neuromodulation techniques: analgesic effects and neural mechanisms]. Sheng Li Xue Bao: [Acta Physiologica Sinica], 73(3), 389–406. https://pubmed.ncbi.nlm.nih.gov/34230942/
Cocchi, L., Zalesky, A., Nott, Z., Whybird, G., Fitzgerald, P. B., & Breakspear, M. (2018). Transcranial magnetic stimulation in obsessive-compulsive disorder: A focus on network mechanisms and state dependence. NeuroImage: Clinical, 19, 661–674. https://doi.org/10.1016/j.nicl.2018.05.029
Del Felice, A., Castiglia, L., Formaggio, E., Cattelan, M., Scarpa, B., Manganotti, P., Tenconi, E., & Masiero, S. (2019). Personalised transcranial alternating current stimulation (tACS) and physical therapy to treat motor and cognitive symptoms in Parkinson’s disease: A randomised cross-over trial. NeuroImage: Clinical, 22, 101768. https://doi.org/10.1016/j.nicl.2019.101768
Eldaief, M. C., Press, D. Z., & Pascual-Leone, A. (2013). Transcranial magnetic stimulation in neurology. Neurology: Clinical Practice, 3(6), 519–526. https://doi.org/10.1212/01.CPJ.0000436213.11132.8e
Elyamany, O., Leicht, G., Herrmann, C. S., & Mulert, C. (2020). Transcranial alternating current stimulation (tACS): from basic mechanisms towards first applications in psychiatry. European Archives of Psychiatry and Clinical Neuroscience, 271(1), 135–156. https://doi.org/10.1007/s00406-020-01209-9
Fakhar, K., Hastings, E., Butson, C. R., Foote, K. D., Zeilman, P., & Okun, M. S. (2013). Management of Deep Brain Stimulator Battery Failure: Battery Estimators, Charge Density, and Importance of Clinical Symptoms. PLoS ONE, 8(3), e58665. https://doi.org/10.1371/journal.pone.0058665
Farcas, A., & Iftene, F. (2022). Findings, limitations and new directions in tACS studies in schizophrenia research: A scoping review. Journal of Psychiatric Research, 151, 291–298. https://doi.org/10.1016/j.jpsychires.2022.04.036
Fick, L. (2016, November 12). Deep Brain Stimulation for Schizophrenia. WebMD. https://www.webmd.com/schizophrenia/deep-brain-stimulation-schizophrenia
Fregni, F., Boggio, P. S., Nitsche, M., & Pascual-Leone, A. (2005). Transcranial direct current stimulation. British Journal of Psychiatry, 186(5), 446–447. https://doi.org/10.1192/bjp.186.5.446
Guleyupoglu, B., Schestatsky, P., Edwards, D., Fregni, F., & Bikson, M. (2013). Classification of methods in transcranial Electrical Stimulation (tES) and evolving strategy from historical approaches to contemporary innovations. Journal of Neuroscience Methods, 219(2), 297–311. https://doi.org/10.1016/j.jneumeth.2013.07.016
Haeckert, J., Lasser, C., Pross, B., Hasan, A., & Strube, W. (2020). Comparative study of motor cortical excitability changes following anodal tDCS or high-frequency tRNS in relation to stimulation duration. Physiological Reports, 8(19), e14595. https://doi.org/10.14814/phy2.14595
Haller, N., Senner, F., Brunoni, A. R., Padberg, F., & Palm, U. (2020). Gamma transcranial alternating current stimulation improves mood and cognition in patients with major depression. Journal of Psychiatric Research, 130, 31–34. https://doi.org/10.1016/j.jpsychires.2020.07.009
Jooss, A., Haberbosch, L., Köhn, A., Rönnefarth, M., Bathe-Peters, R., Kozarzewski, L., Fleischmann, R., Scholz, M., Schmidt, S., & Brandt, S. A. (2019). Motor Task-Dependent Dissociated Effects of Transcranial Random Noise Stimulation in a Finger-Tapping Task Versus a Go/No-Go Task on Corticospinal Excitability and Task Performance. Frontiers in Neuroscience, 13. https://doi.org/10.3389/fnins.2019.00161
Joseph, B. (2015). Indian Act and the Pass System. Www.ictinc.ca. https://www.ictinc.ca/blog/indian-act-and-the-pass-system
Klas, N. (2022). Biological age diagnostics report. Longevity Technology Reports. https://longevity.technology/research/report/biological-age-diagnostics-report/
Krames, E. S., Peckham, H., Rezai, A., & Aboelsaad, F. (2009). Chapter 1 – what is neuromodulation? (E. S. Krames, P. P. Hunter, & A. R. Rezai, Eds.). ScienceDirect; Academic Press. https://www.sciencedirect.com/science/article/pii/B9780123742483000021#:~:text=The%20term%20neuromodulation%20can%20be%20defined%20as%20a
Krauss, J. K., Lipsman, N., Aziz, T., Boutet, A., Brown, P., Chang, J. W., Davidson, B., Grill, W. M., Hariz, M. I., Horn, A., Schulder, M., Mammis, A., Tass, P. A., Volkmann, J., & Lozano, A. M. (2020). Technology of deep brain stimulation: current status and future directions. Nature Reviews Neurology, PMC7116699(33244188), 1–13. https://doi.org/10.1038/s41582-020-00426-z
Montgomery, E. B., & Gale, J. T. (2008). Mechanisms of action of deep brain stimulation (DBS). Neuroscience & Biobehavioral Reviews, 32(3), 388–407. https://doi.org/10.1016/j.neubiorev.2007.06.003
Moret, B., Camilleri, R., Pavan, A., Lo Giudice, G., Veronese, A., Rizzo, R., & Campana, G. (2018). Differential effects of high-frequency transcranial random noise stimulation (HF-tRNS) on contrast sensitivity and visual acuity when combined with a short perceptual training in adults with amblyopia. Neuropsychologia, 114, 125–133. https://doi.org/10.1016/j.neuropsychologia.2018.04.017
Moret, B., Donato, R., Nucci, M., Cona, G., & Campana, G. (2019). Transcranial random noise stimulation (tRNS): a wide range of frequencies is needed for increasing cortical excitability. Scientific Reports, 9(1). https://doi.org/10.1038/s41598-019-51553-7
Nafise, S. N., Minoo, K. Z., & Khosro, K. (2016). Effects and side effects of tDCS in movement disorders of children and adolescents. Undefined. https://www.semanticscholar.org/paper/EFFECTS-AND-SIDE-EFFECTS-OF-TDCS-IN-MOVEMENT-OF-AND-Nafise-Minoo/45b9f656c665b7169814cf9cdb796509b2aba916
Naidech, A. M. (2011). Intracranial Haemorrhage. American Journal of Respiratory and Critical Care Medicine, 184(9), 998–1006. https://doi.org/10.1164/rccm.201103-0475ci
Nitsche, M. A., Cohen, L. G., Wassermann, E. M., Priori, A., Lang, N., Antal, A., Paulus, W., Hummel, F., Boggio, P. S., Fregni, F., & Pascual-Leone, A. (2008). Transcranial direct current stimulation: State of the art 2008. Brain Stimulation, 1(3), 206–223. https://doi.org/10.1016/j.brs.2008.06.004
Peng, L., Fu, J., Ming, Y., Zeng, S., He, H., & Chen, L. (2018). The long-term efficacy of STN vs GPi deep brain stimulation for Parkinson disease. Medicine, 97(35), e12153. https://doi.org/10.1097/md.0000000000012153
Potok, W., Bächinger, M., van der Groen, O., Cretu, A. L., & Wenderoth, N. (2021). Transcranial Random Noise Stimulation Acutely Lowers the Response Threshold of Human Motor Circuits. The Journal of Neuroscience, 41(17), 3842–3853. https://doi.org/10.1523/JNEUROSCI.2961-20.2021
Rapinesi, C., Kotzalidis, G. D., Ferracuti, S., Sani, G., Girardi, P., & Del Casale, A. (2019). Brain Stimulation in Obsessive-Compulsive Disorder (OCD): A Systematic Review. Current Neuropharmacology, 17. https://doi.org/10.2174/1570159×17666190409142555
Reed, T., & Cohen Kadosh, R. (2018). Transcranial electrical stimulation (tES) mechanisms and its effects on cortical excitability and connectivity. Journal of Inherited Metabolic Disease, 41(6), 1123–1130. https://doi.org/10.1007/s10545-018-0181-4
Rossi, M., Bruno, V., Arena, J., Cammarota, Á., & Merello, M. (2018). Challenges in PD Patient Management After DBS: A Pragmatic Review. Movement Disorders Clinical Practice, 5(3), 246–254. https://doi.org/10.1002/mdc3.12592
Scheper, A., Rosenfeld, C., & Dubljević, V. (2022). The public impact of academic and print media portrayals of TMS: shining a spotlight on discrepancies in the literature. BMC Medical Ethics, 23(1). https://doi.org/10.1186/s12910-022-00760-5
Shiozawa, P., Fregni, F., Benseñor, I., Lotufo, P., Berlim, M., Daskalakis, J., Cordeiro, Q., & Brunoni, A. (2014). Transcranial direct current stimulation for major depression: an updated systematic review and meta-analysis. Undefined. https://www.semanticscholar.org/paper/Transcranial-direct-current-stimulation-for-major-Shiozawa-Fregni/a3b01b64fcdd6a4c087120bb4d891358fe217006
Shobo, M., Yamada, H., Koakutsu, A., Hamada, N., Fujii, M., Harada, K., Ni, K., & Matsuoka, N. (2011). Chronic treatment with olanzapine via a novel infusion pump induces adiposity in male rats. Life Sciences, 88, 761–765. https://doi.org/10.1016/j.lfs.2011.02.014
Spielberg, B. (2020, August 18). The Real Pros and Cons of TMS Therapy. TMS & Brain Health. https://www.tmsbrainhealth.com/the-real-pros-and-cons-of-tms-therapy/
Sprengers, M., Vonck, K., Carrette, E., Marson, A.G., & Boon, P.M, S., K, V., E, C., Ag, M., & P, B. (2014). Deep brain and cortical stimulation for epilepsy. The Cochrane Database of Systematic Reviews, 24937707. https://doi.org/10.1002/14651858.CD008497.pub2
Terney, D., Chaieb, L., Moliadze, V., Antal, A., & Paulus, W. (2008). Increasing Human Brain Excitability by Transcranial High-Frequency Random Noise Stimulation. Journal of Neuroscience, 28(52), 14147–14155. https://doi.org/10.1523/jneurosci.4248-08.2008
Zorzo, C., Banqueri, M., Higarza, S. G., Pernía, A. M., & Arias, J. L. (2019). Current State of Transcranial Magnetic Stimulation and its use in Psychiatry. Actas Espanolas de Psiquiatria, 47(3), 110–120. https://europepmc.org/article/med/31233209